- South Africa
- View all news
- Agribusiness
- Empowerment
- Tax & Management
- View all business
- Sheep & Goats
- Game & Wildlife
- Aquaculture
- view all animals
- Fruit & Nuts
- view all crops
- Machinery & Equipment
- Farming for Tomorrow
- How to: Business
- How to: Crop
- How to: Livestock
- View all farming basics
- Agritourism
- Farms for sale
- View Classifieds
- Place an Ad
- Request a quote
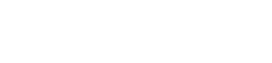

The importance of research in agriculture
The eighth episode of tech terrain deliberates over the role research plays in agriculture and how it can increase productivity to meet the food demand of the world..
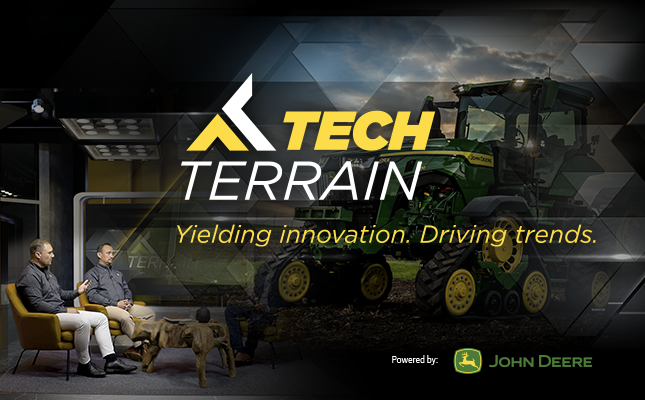
Since the start of the agricultural revolution, the sector has been defined by research and innovation, which include technology development that is adopted throughout the value chain, comprehensive and inclusive of digital solutions.
And this also includes the very important topic of ethical and safe practices – both for industry role players, consumers and the environment.
In this week’s episode of Tech Terrain, Tony Ndoro talks to two distinguished academics – Charlie Reinhardt, Professor of Agronomy, Faculty of Agriculture: North-West University and Prof Lise Korsten of the Department of Plant and Soil Sciences at the University of Pretoria to share their views on the value of research for the sector.
And later on in the episode, Matome Ramokgopa, Managing Director: Enza Zaden South Africa discusses the application of agricultural research on the African continent.
A word from John Deere
You can also look forward to a discussion about the John Deere 6M tractor with Admire Mutsvairo, Business Operations Analyst for John Deere Sub-Saharan Africa (JD SSA) and Hein Snyman, Territory Sales Manager for JD SSA.
Visit techterrain.co.za to get access to all the content plus bonus material. New episodes exploring new and relevant themes will be released every Thursday at 16:00pm.
Powered by John Deere, in collaboration with Farmer’s Weekly and Brand Republic.
MORE FROM FARMERS WEEKLY
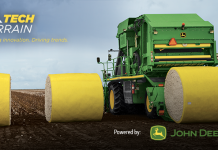
Episode 16: The value of cotton
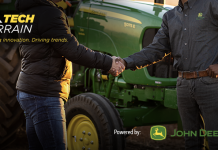
Episode 15: The economy and agricultural financing
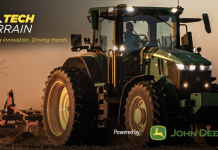
The good farmers do
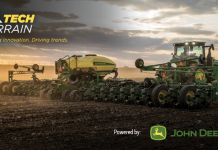
Episode 13: High-value export commodities
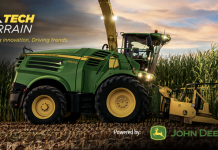
Episode 12 – Conservation Agriculture
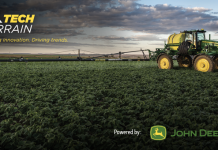
Episode 11: The increasing importance of biosecurity

Interest rate drops marginally, but signals better times ahead
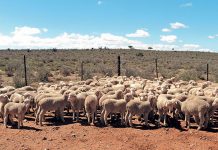
Understand livestock pricing structures to avoid financial losses

Agribusiness confidence rebounds despite ongoing challenges
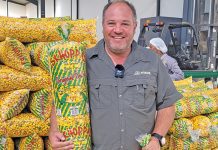
Award-winning farmer achieves success through diversified business

How important is a brand name in modern agriculture?
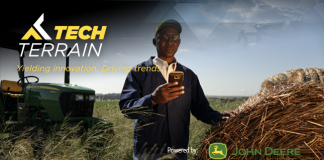
Farming optimally and getting the most out of your land
Agruculture Lore
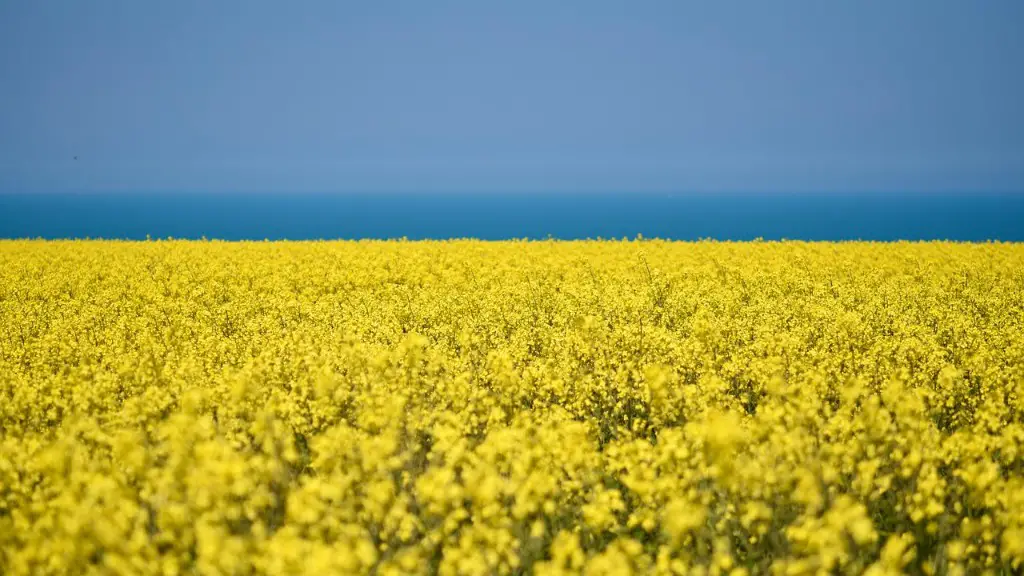
Why Is Research Important In Agriculture
Research has become an indispensable part of modern agriculture. It is used to solve various problems faced by the agricultural sector, from pest control to land management. Research helps farmers stay ahead of the curve, allowing them to make better decisions, use up-to-date information and make the most out of their crops and land. It’s also essential in understanding the changing climate and developing better strategies for facing challenges brought about by weather conditions.
Research in agriculture helps in the development of new methods and techniques of farming, some of which are more efficient, cost-effective, and ecologically beneficial. These new techniques, if implemented in the right way, can help increase the productivity of a farm and lower the risk to crops. Newer methods are also required in order to tackle the ever-changing pest infestations, soil erosion and other threats.
Moreover, research helps identify suitable crops for particular soils and climates, and lets farmers know which fertilizers and insecticides should be used in order to yield better crops. Research is also necessary for the development of innovative and eco-friendly farming practices, such as more efficient irrigation systems, crop rotation, and sustainable management of natural resources.
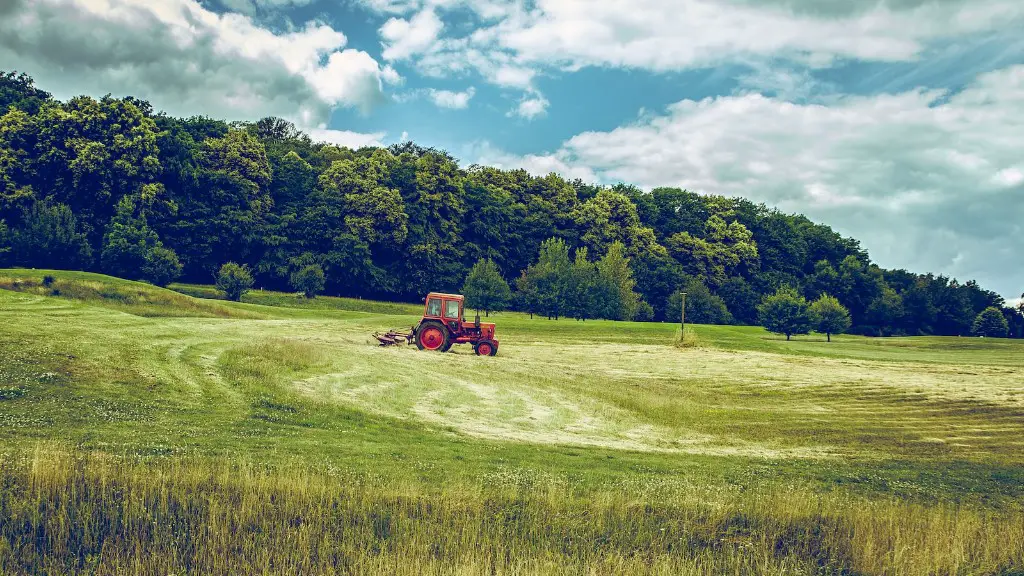
Studies are imperative for efficient farm management, as they can provide farmers and agronomists with important indicators about the status of their crops and land. For example, research can help better identify the factors that can influence the growth of crops, such as temperature, soil composition, and amount of sunlight. With this data, farmers can make calculated decisions to maximize their yield and minimize crop damage, such as selecting the best time to sow, irrigate, and harvest.
Furthermore, research in agriculture can help to preserve and improve the quality of life of those who depend on it. Studies have shown that research-based improvements in agricultural methods can lead to higher incomes for farmers and other members of the agricultural workforce. Research has also identified innovative approaches for improving nutrition, which is especially beneficial for enhancing the quality of life in rural communities.
As you can see, research is extremely important in agriculture. It’s essential for the development of efficient and profitable farming methods, as well as for preserving and improving the quality of life of those who depend on farming as their livelihood.
Current trends in agricultural research
At present, agricultural research has advanced to encompass new technologies, such as the use of satellite imagery, advanced agricultural software, and precision farming. Data collected through research is used to better understand the factors that influence the growth and health of crops. This data is also analyzed to identify areas for improvement, such as the optimal arrangement of crops, or the appropriate use of fertilizers.
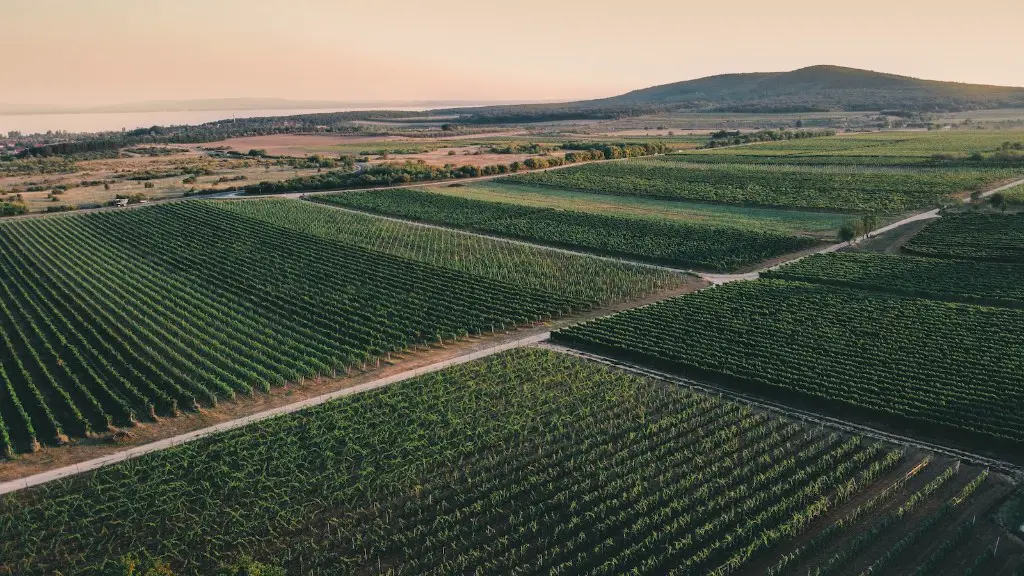
Furthermore, research is also used to investigate and develop beneficial training and educational opportunities for farmers. Research findings aid agricultural organizations in developing more effective methods for promoting the modern practices of farming. The use of information gleaned from research is also critical for the success of agricultural programs implemented by the government.
In addition to these areas of research, more resources are also being devoted to studying the various genetic components involved in crop production and environmental sustainability. This involves researching and developing new genetic material, as well as gene manipulation tactics. The potential of this kind of research is massive, as it could enable farmers to produce entirely new hybrid varieties of plants, specifically designed to resist certain pests or harsh climatic conditions.
Movements like organic agriculture are further expanding the scope of agricultural research, as a lot of studies are conducted to explore new agro-ecological methods and to understand their environmental, economic, and health benefits.
The impact of agricultural research
The impact of agricultural research is felt in every aspect of agricultural life, from the use of ingenious techniques to boost production to the implementation of improved safety protocols. The use of research-based findings can lead to more efficient cultivation methods and improved product yield.
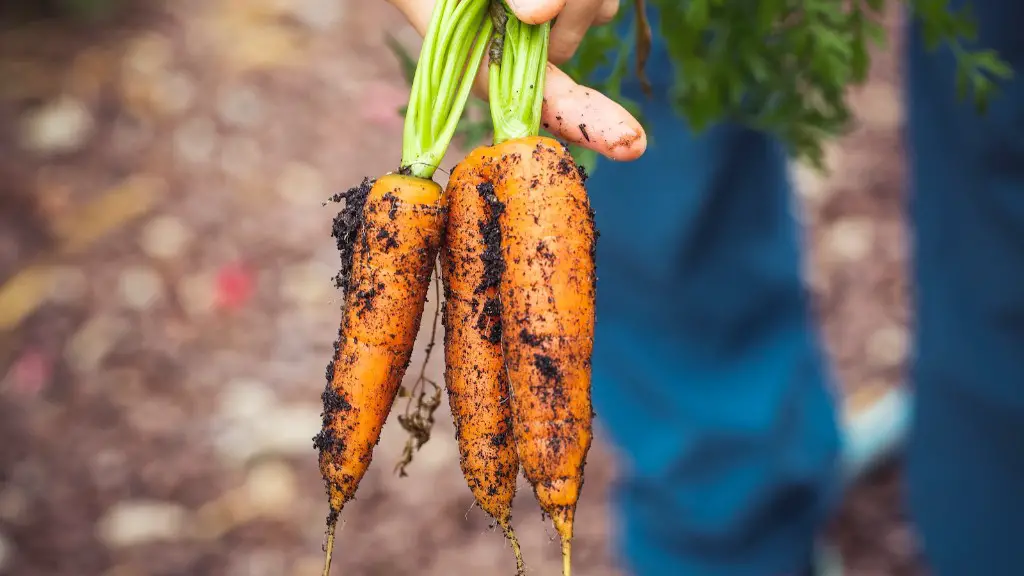
At the same time, the use of research-based information also helps to minimize risks to farmers and their crops. With access to the right information and resources, farmers can better understand and combat environmental threats and make sound decisions when it comes to preparing fields and crops.
Agricultural research also helps advance food security initiatives, as it can provide us with the data needed to identify nutritious crops and healthy livestock breeds that can help feed a growing global population. Research also enables us to expand our knowledge about the nature of agricultural products and the ways in which we can best use them.
The advancements made in research have also modernized agricultural methods and processes, with studies leading to the introduction of sophisticated machines and equipment that are efficient, reliable, and controlled by smart technologies. Automation is being increasingly used to monitor and manage crop production, from the moment of sowing to the time of harvest. Research-based solutions also enable farmers to reduce labor costs and save resources.
The potential of agricultural research
Agricultural research is essential for maintaining our access to food and other resources. As such, research plays a significant role in global efforts to achieve food security, a sustainable environment, and a healthier population.
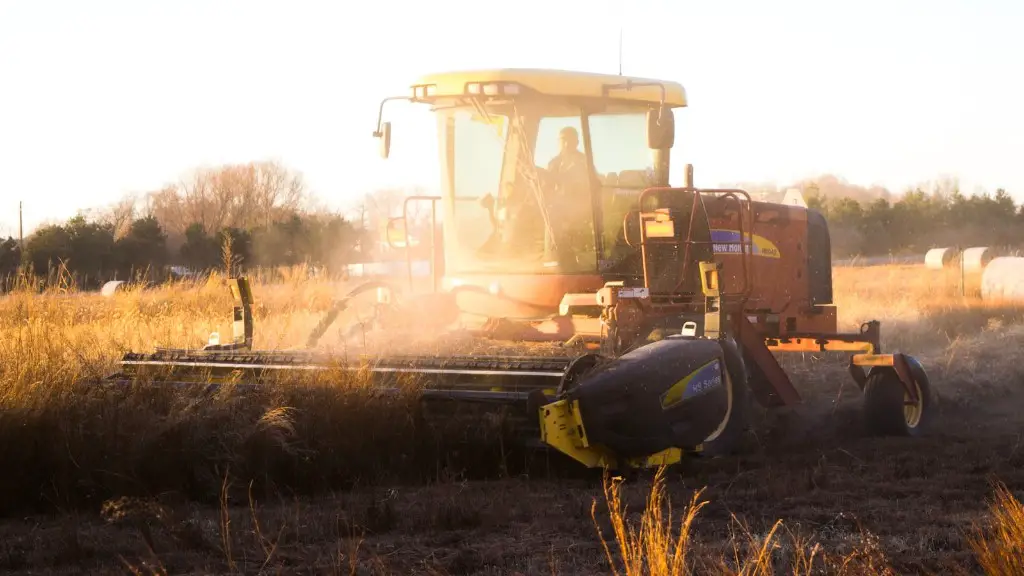
As the world’s population and demand for food continue to increase, it is essential that we not only focus our attention on optimizing existing methods, but also direct resources towards discovering new and better ones. Moreover, research is essential for mapping out the ways in which we can help protect our planet for future generations.
Although the application of research-based solutions is still in its early stages in many parts of the world, it can already have a massive positive impact. For example, studies have already identified the use of certain plants as a form of pest control, which could have a huge impact on reducing the use of insecticides, herbicides, and fungicides.
Research has also been instrumental in helping create healthier crops and livestock, as studies have shown that it is possible to develop and promote varieties that are resistant to certain diseases and pests. This could help farmers reduce their crop losses and, in turn, improve their incomes.
Research is an indispensable tool in modern agriculture, as it is the key in helping us advance in the areas like pest control, land management, developing new crops, and improving farm management. As our technology rapidly advances, research is also essential in monitoring, customizing, and improving agricultural methods to better serve the world’s population. Additionally, agricultural research might be the catalyst in enabling us to create a sustainable, healthy, and equitable global food system.
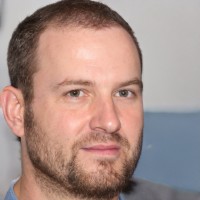
Eduardo Villanueva
Eduardo Villanueva is an expert on agricultural sciences, with decades of experience in the field. With a passion for teaching others, Eduardo has written extensively about topics related to sustainable agriculture and food security. His work aims to empower rural farmers and promote responsible farming practices that help preserve the environment for future generations. A dedicated family man, Eduardo lives in central Mexico with his wife and children. He is always looking for ways to connect people and knowledge to create positive changes in their local communities.
Leave a Comment Cancel reply

- My Favorites
You have successfully logged in but...
... your login credentials do not authorize you to access this content in the selected format. Access to this content in this format requires a current subscription or a prior purchase. Please select the WEB or READ option instead (if available). Or consider purchasing the publication.
OECD Food, Agriculture and Fisheries Papers
The benefits from agricultural research and development, innovation, and productivity growth.
NB. No. 1 to No. 58 were released under the previous series title OECD Food, Agriculture and Fisheries Working Papers .

- Agriculture and Food
- ISSN: 18156797 (online)
- https://doi.org/10.1787/18156797
- Subscribe to the RSS feed Subscribe to the RSS feed
English Also available in: French
- Click to access:
- Click to download PDF - 424.61KB PDF

Cite this content as:
Author(s) Julian M. Alston i i University of California
01 Aug 2010
- Search Menu
- Sign in through your institution
- Advance articles
- Author Guidelines
- Submission Site
- Open Access
- Why Publish?
- About Research Evaluation
- Editorial Board
- Advertising and Corporate Services
- Journals Career Network
- Self-Archiving Policy
- Dispatch Dates
- Journals on Oxford Academic
- Books on Oxford Academic

Article Contents
1. introduction, 2. analytical framework, 3. literature search, 5. discussion, 6. conclusion, acknowledgement.
- < Previous
Research impact assessment in agriculture—A review of approaches and impact areas
- Article contents
- Figures & tables
- Supplementary Data
Peter Weißhuhn, Katharina Helming, Johanna Ferretti, Research impact assessment in agriculture—A review of approaches and impact areas, Research Evaluation , Volume 27, Issue 1, January 2018, Pages 36–42, https://doi.org/10.1093/reseval/rvx034
- Permissions Icon Permissions
Research has a role to play in society’s endeavour for sustainable development. This is particularly true for agricultural research, since agriculture is at the nexus between numerous sustainable development goals. Yet, generally accepted methods for linking research outcomes to sustainability impacts are missing. We conducted a review of scientific literature to analyse how impacts of agricultural research were assessed and what types of impacts were covered. A total of 171 papers published between 2008 and 2016 were reviewed. Our analytical framework covered three categories: (1) the assessment level of research (policy, programme, organization, project, technology, or other); (2) the type of assessment method (conceptual, qualitative, or quantitative); and (3) the impact areas (economic, social, environmental, or sustainability). The analysis revealed that most papers (56%) addressed economic impacts, such as cost-effectiveness of research funding or macroeconomic effects. In total, 42% analysed social impacts, like food security or aspects of equity. Very few papers (2%) examined environmental impacts, such as climate effects or ecosystem change. Only one paper considered all three sustainability dimensions. We found a majority of papers assessing research impacts at the level of technologies, particularly for economic impacts. There was a tendency of preferring quantitative methods for economic impacts, and qualitative methods for social impacts. The most striking finding was the ‘blind eye’ towards environmental and sustainability implications in research impact assessments. Efforts have to be made to close this gap and to develop integrated research assessment approaches, such as those available for policy impact assessments.
Research has multiple impacts on society. In the light of the international discourse on grand societal challenges and sustainable development, the debate is reinforced about the role of research on economic growth, societal well-being, and environmental integrity ( 1 ). Research impact assessment (RIA) is a key instrument to exploring this role ( 2 ).
A number of countries have begun using RIA to base decisions for allocation of funding on it, and to justify the value of investments in research to taxpayers ( 3 ). The so-called scientometric assessments with a focus on bibliometric and exploitable results such as patents are the main basis for current RIA practices ( 4–6 ). However, neither academic values of science, based on the assumption of ‘knowledge as progress’, nor market values frameworks (‘profit as progress’) seem adequate for achieving and assessing broader public values ( 7 ). Those approaches do not explicitly acknowledge the contribution of research to solving societal challenges, although they are sufficient to measure scientific excellence ( 8 ) or academic impact.
RIA may however represent a vital element for designing socially responsible research processes with orientation towards responsibility for a sustainable development ( 9 , 10 ). In the past, RIAs occurred to focus on output indicators and on links between science and productivity while hardly exploring the wider societal impacts of science ( 11 ). RIA should entail the consideration of intended and non-intended, positive and negative, and long- and short-term impacts of research ( 12 ). Indeed, there has been a broadening of impact assessments to include, for example, cultural and social returns to society ( 13 ). RIA is conceptually and methodologically not yet sufficiently equipped to capture wider societal implications, though ( 14 ). This is due to the specific challenges associated with RIA, including inter alia unknown time lags between research processes and their impacts ( 15–17 ). Independent from their orientation, RIAs are likely to influence research policies for years to come ( 18 ).
Research on RIA and its potential to cover wider societal impacts has examined assessment methods and approaches in specific fields of research, and in specific research organizations. The European Science Foundation ( 19 ) and Guthrie et al. ( 20 ) provided overviews of a range of methods usable in assessment exercises. They discuss generic methods (e.g. economic analyses, surveys, and case studies) with view to their selection for RIAs. Methods need to fit the objectives of the assessment and the characteristics of the disciplines examined. Econometric methods consider the rate of return over investment ( 21 ), indicators for ‘productive interactions’ between the stakeholders try to capture the social impact of research ( 22 ), and case study-based approaches map the ‘public values’ of research programmes ( 8 , 23 ). No approach is generally favourable over another, while challenges exist in understanding which impact areas are relevant in what contexts. Penfield et al. ( 6 ) looked at the different methods and frameworks employed in assessment approaches worldwide, with a focus on the UK Research Excellence Framework. They argue that there is a need for RIA approaches based on types of impact rather than research discipline. They point to the need for tools and systems to assist in RIAs and highlight different types of information needed along the output-outcome-impact-chain to provide for a comprehensive assessment. In the field of public health research, a minority of RIAs exhibit a wider scope on impacts, and these studies highlight the relevance of case studies ( 24 ). However, case studies often rely on principal investigator interviews and/or peer review, not taking into account the views of end users. Evaluation practices in environment-related research organizations tend to focus on research uptake and management processes, but partially show a broader scope and longer-term outcomes. Establishing attribution of environmental research to different types of impacts was identified to be a key challenge ( 25 ). Other authors tested impact frameworks or impact patterns in disciplinary public research organizations. For example, Gaunand et al. ( 26 ) analysed an internal database of the French Agricultural research organization INRA with 1,048 entries to identify seven impact areas, with five going beyond traditional types of impacts (e.g. conservation of natural resources or scientific advice). Besides, for the case of agricultural research, no systematic review of RIA methods exists in the academic literature that would allow for an overview of available approaches covering different impact areas of research.
Against this background, the objective of this study was to review in how far RIAs of agricultural research capture wider societal implications. We understand agricultural research as being a prime example for the consideration of wider research impacts. This is because agriculture is a sector which has direct and severe implications for a range of the UN Sustainable Development Goals. It has a strong practice orientation and is just beginning to develop a common understanding of innovation processes ( 27 ).
The analysis of the identified literature on agricultural RIA (for details, see next section ‘Literature search’) built on a framework from a preliminary study presented at the ImpAR Conference 2015 ( 28 ). It was based on three categories to explore the impact areas that were addressed and the design of RIA. In particular, the analytical framework consisted of: ( 1 ) the assessment level of research; ( 2 ) the type of assessment method; and ( 3 ) the impact areas covered. On the side, we additionally explored the time dimension of RIA, i.e. whether the assessment was done ex ante or ex post (see Fig. 1 ).

Analytical framework for the review of non-scientometric impact assessment literature of agricultural research.
Agricultural research and the ramifications following from that refer to different levels of assessment (or levels of evaluation, ( 29 )). We defined six assessment levels that can be the subject of a RIA: policy, programme, organization, project, technology, and other. The assessment level of the RIA is a relevant category, since it shapes the approach to the RIA (e.g. the impact chain of a research project differs to that at policy level). The assessment level was clearly stated in all of the analysed papers and in no case more than one assessment level was addressed. Articles were assigned to the policy level, if a certain public technology policy ( 30 ) or science policy, implemented by governments to directly or indirectly affect the conduct of science, was considered. Exemplary topics are research funding, transfer of research results to application, or contribution to economic development. Research programmes were understood as instruments that are adopted by government departments, or other organizational entities to implement research policies and fund research activities in a specific research field (e.g. programmes to promote research on a certain crop or cultivation technique). Articles dealing with the organizational level assess the impact of research activities of a specific research organization. The term research organization comprises public or private research institutes, associations, networks, or partnerships (e.g. the Consultative Group on International Agricultural Research (CGIAR) and its research centres). A research project is the level at which research is actually carried out, e.g. as part of a research programme. The assessment of a research project would consider the impacts of the whole project, from planning through implementation to evaluation instead of focusing on a specific project output, like a certain agricultural innovation. The technology level was considered to be complementary to the other assessment levels of research and comprises studies with a strong focus on specific agricultural machinery or other agricultural innovation such as new crops or crop rotations, fertilizer applications, pest control, or tillage practices, irrespective of the agricultural system (e.g. smallholder or high-technology farming, or organic, integrated, or conventional farming). The category ‘other’ included one article addressing RIA at the level of individual researchers (see ( 31 )).
We categorized the impact areas along the three dimensions of sustainable development by drawing upon the European Commission’s impact assessment guidelines (cf. ( 32 )). The guidelines entail a list of 7 environmental impacts, such as natural resource use, climate change, or aspects of nature conservation; 12 social impacts, such as employment and working conditions, security, education, or aspects of equity; and 10 economic impacts, including business competitiveness, increased trade, and several macroeconomic aspects. The European Commission’s impact assessment guidelines were used as a classification framework because it is one of the most advanced impact assessment frameworks established until to date ( 33 ). In addition, we opened a separate category for those articles exploring joint impacts on the three sustainability dimensions. Few articles addressed impacts in two sustainability dimensions which we assigned to the dominating impact area.
To categorize the type of RIA method, we distinguished between conceptual, qualitative, and quantitative. Conceptual analyses include the development of frameworks or concepts for measuring impacts of agricultural research (e.g. tracking of innovation pathways or the identification of barriers and supporting factors for impact generation). Qualitative and quantitative methods were identified by the use of qualitative data or quantitative data, respectively (cf. ( 34–36 )). Qualitative data can be scaled nominally or ordinally. It is generated by interviews, questionnaires, surveys or choice experiments to gauge stakeholder attitudes to new technologies, their willingness to pay, and their preference for adoption measures. The generation of quantitative data involves a numeric measurement in a standardized way. Such data are on a metric scale and are often used for modelling. The used categorization is rather simple. We assigned approaches which employed mixed-method approaches according to their dominant method. We preferred this over more sophisticated typologies to achieve a high level of abstraction and because the focus of our analysis was on impact areas rather than methods. However, to show consistencies with existing typologies of impact assessment methods ( 19 , 37 ), we provide an overview of the categorization chosen and give examples of the most relevant types of methods.
To additionally explore the approach of the assessment ( 38 ), the dimensions ex ante and ex post were identified. The two approaches are complementary: whereas ex ante impact assessments are usually conducted for strategic and planning purposes to set priorities, ex post impact assessments serve as accountability validation and control against a baseline. The studies in our sample that employed an ex ante approach to RIA usually made this explicit, while in the majority of ex post impact assessments, this was indicated rather implicitly.
This study was performed as a literature review based on Thomson Reuters Web of Science TM Core Collection, indexed in the Science Citation Index Expanded (SCI-Exp) and the Social Sciences Citation Index (SSCI). The motivation for restricting the analysis to articles from ISI-listed journals was to stay within the boundaries of internationally accepted scientific quality management and worldwide access. The advantages of a search based on Elsevier’s Scopus ® (more journals and alternative publications, and more articles from social and health science covered) would not apply for this literature review, with regard to the drawbacks of an index system based on abstracts instead of citation indexes, which is not as transparent as the Core Collection regarding the database definable by the user. We selected the years of 2008 to mid-2016 for the analysis (numbers last updated on 2 June 2016) . First, because most performance-based funding systems have been introduced since 2000, allowing sufficient time for the RIA approaches to evolve and literature to be published. Secondly, in 2008 two key publications on RIA of agricultural research triggered the topic: Kelley, et al. ( 38 ) published the lessons learned from the Standing Panel on Impact Assessment of CGIAR; Watts, et al. ( 39 ) summarized several central pitfalls of impact assessment concerning agricultural research. We took these publications as a starting point for the literature search. We searched in TOPIC and therefore, the terms had to appear in the title, abstract, author keywords, or keywords plus ® . The search query 1 filtered for agricultural research in relation to research impact. To cover similar expressions, we used science, ‘R&D’, and innovation interchangeably with research, and we searched for assessment, evaluation, criteria, benefit, adoption, or adaptation of research.
We combined the TOPIC search with a less strict search query 2 in TITLE using the same groups of terms, as these searches contained approximately two-thirds non-overlapping papers. Together they consisted of 315 papers. Of these, we reviewed 282 after excluding all document types other than articles and reviews (19 papers were not peer-reviewed journal articles) and all papers not written in English language (14 papers). After going through them, 171 proved to be topic-relevant and were included in the analysis.
Analysis matrix showing the number of reviewed articles, each categorized to an assessment level and an impact area (social, economic, environmental, or all three (sustainability)). Additionally, the type of analytical method (conceptual, quantitative, and qualitative) is itemized
Assessment level . | Policy . | Programme . | Organization . | Project . | Technology . | Others . | Sum . |
---|---|---|---|---|---|---|---|
Impact area . | |||||||
Social issues | |||||||
Conceptual | 7 | 3 | 1 | 4 | 6 | 0 | 21 |
Qualitative | 6 | 4 | 4 | 10 | 11 | 0 | 35 |
Quantitative | 5 | 2 | 5 | 2 | 2 | 0 | 16 |
Economy | |||||||
Conceptual | 3 | 4 | 6 | 5 | 13 | 1 | 32 |
Qualitative | 2 | 2 | 1 | 3 | 19 | 0 | 27 |
Quantitative | 8 | 6 | 4 | 4 | 14 | 0 | 36 |
Environment | |||||||
Conceptual | 0 | 0 | 0 | 0 | 1 | 0 | 1 |
Qualitative | 0 | 0 | 0 | 1 | 0 | 0 | 1 |
Quantitative | 0 | 0 | 0 | 0 | 1 | 0 | 1 |
Sustainability | |||||||
Conceptual | 0 | 0 | 0 | 0 | 1 | 0 | 1 |
Qualitative | 0 | 0 | 0 | 0 | 0 | 0 | 0 |
Quantitative | 0 | 0 | 0 | 0 | 0 | 0 | 0 |
Total |
Assessment level . | Policy . | Programme . | Organization . | Project . | Technology . | Others . | Sum . |
---|---|---|---|---|---|---|---|
Impact area . | |||||||
Social issues | |||||||
Conceptual | 7 | 3 | 1 | 4 | 6 | 0 | 21 |
Qualitative | 6 | 4 | 4 | 10 | 11 | 0 | 35 |
Quantitative | 5 | 2 | 5 | 2 | 2 | 0 | 16 |
Economy | |||||||
Conceptual | 3 | 4 | 6 | 5 | 13 | 1 | 32 |
Qualitative | 2 | 2 | 1 | 3 | 19 | 0 | 27 |
Quantitative | 8 | 6 | 4 | 4 | 14 | 0 | 36 |
Environment | |||||||
Conceptual | 0 | 0 | 0 | 0 | 1 | 0 | 1 |
Qualitative | 0 | 0 | 0 | 1 | 0 | 0 | 1 |
Quantitative | 0 | 0 | 0 | 0 | 1 | 0 | 1 |
Sustainability | |||||||
Conceptual | 0 | 0 | 0 | 0 | 1 | 0 | 1 |
Qualitative | 0 | 0 | 0 | 0 | 0 | 0 | 0 |
Quantitative | 0 | 0 | 0 | 0 | 0 | 0 | 0 |
Total |
In the agricultural RIA, the core assessment level of the reviewed articles was technology (39%), while the other levels were almost equally represented (with the exception of ‘other’). Generally, most papers (56%) addressed economic research impacts, closely followed by social research impacts (42%); however, only three papers (2%) addressed environmental research impacts and only 1 of 171 papers addressed all three dimensions of sustainable development. Assessments at the level of research policy slightly emphasized social impacts over economic impacts (18 papers, or 58%), whereas assessments at the level of technology clearly focused primarily on economic impacts (46 papers, or 68%).
The methods used for agricultural RIA showed no preference for one method type (see Table 1 ). Approximately 31% of the papers assessed research impacts quantitatively, whereas 37% used qualitative methods. Conceptual considerations on research impact were applied by 32% of the studies. A noticeable high number of qualitative studies were conducted to assess social impacts. At the evaluation level of research policy and research programmes, we found a focus on quantitative methods, if economic impacts were assessed.
Overview on type of methods used for agricultural RIA
Method Type I . | Method Type II . | Example . |
---|---|---|
Conceptual | Review | Document analysis, literature review, argumentation, anecdotes |
Framework development | Conceptual innovation | |
Qualitative | Survey | Questionnaire, interview, expert surveys, etc. |
Quantitative | Stochastic method | Regression analysis, Bayesian probabilistic method |
Economic valuation | Econometric analysis, cost–benefit analysis, cost-effectiveness | |
Mixed | Participatory evaluation | Individual rating, group voting, actor mapping, evaluation of assessment tools |
Case studies | Detailed analysis of individual research projects, programmes, etc. |
Method Type I . | Method Type II . | Example . |
---|---|---|
Conceptual | Review | Document analysis, literature review, argumentation, anecdotes |
Framework development | Conceptual innovation | |
Qualitative | Survey | Questionnaire, interview, expert surveys, etc. |
Quantitative | Stochastic method | Regression analysis, Bayesian probabilistic method |
Economic valuation | Econometric analysis, cost–benefit analysis, cost-effectiveness | |
Mixed | Participatory evaluation | Individual rating, group voting, actor mapping, evaluation of assessment tools |
Case studies | Detailed analysis of individual research projects, programmes, etc. |
a Mix of conceptual and qualitative methods.
b Mix of conceptual, qualitative, and quantitative methods.
Additionally, 37 ex ante studies, compared to 134 ex post studies, revealed that the latter clearly dominated, but no robust relation to any other investigated characteristic was found. Of the three environmental impact studies, none assessed ex ante , while the one study exploring sustainability impacts did. The share of ex ante assessments regarding social impacts was very similar to those regarding economic impacts. Within the assessment levels of research (excluding ‘others’ with only one paper), no notable difference between the shares of ex ante assessments occurred as they ranged between 13 and 28%.
The most relevant outcome of the review analysis was that only 3 of the 171 papers focus on the environmental impacts of agricultural research. This seems surprising because agriculture is dependent on an intact environment. However, this finding is supported by two recent reviews: one from Bennett, et al. ( 40 ) and one from Maredia and Raitzer ( 41 ). Both note that not only international agricultural research in general but also research on natural resource management shows a lack regarding large-scale assessments of environmental impacts. The CGIAR also recognized the necessity to deepen the understanding of the environmental impacts of its work because RIAs had largely ignored environmental benefits ( 42 ).
A few papers explicitly include environmental impacts of research in addition to their main focus. Raitzer and Maredia ( 43 ) address water depletion, greenhouse gas emissions, and landscape effects; however, their overall focus is on poverty reduction. Ajayi et al. ( 44 ) report the improvement of soil physical properties and soil biodiversity from introducing fertilizer trees but predominantly measure economic and social effects. Cavallo, et al. ( 45 ) investigate users’ attitudes towards the environmental impact of agricultural tractors (considered as technological innovation) but do not measure the environmental impact. Briones, et al. ( 46 ) configure an environmental ‘modification’ of economic surplus analysis, but they do not prioritize environmental impacts.
Of course, the environmental impacts of agricultural practices were the topic of many studies in recent decades, such as Kyllmar, et al. ( 47 ), Skinner, et al. ( 48 ), Van der Werf and Petit ( 49 ), among many others. However, we found very little evidence for the impact of agricultural research on the environment. A study on environmental management systems that examined technology adoption rates though not the environmental impacts is exemplarily for this ( 50 ). One possible explanation is based on the observation made by Morris, et al. ( 51 ) and Watts, et al. ( 39 ). They see impact assessments tending to accentuate the success stories because studies are often commissioned strategically as to demonstrate a certain outcome. This would mean to avoid carving out negative environmental impacts that conflict with, when indicated, the positive economic or societal impacts of the assessed research activity. In analogy to policy impact assessments, this points to the need of incentives to equally explore intended and unintended, expected and non-expected impacts from scratch ( 52 ). From those tasked with an RIA, this again requires an open attitude in ‘doing RIA’ and towards the findings of their RIA.
Another possible explanation was given by Bennett, et al. ( 40 ): a lack of skills in ecology or environmental economics to cope with the technically complex and data-intensive integration of environmental impacts. Although such a lack of skills or data could also apply to social and economic impacts, continuous monitoring of environmental data related to agricultural practices is particularly scarce. A third possible explanation is a conceptual oversight, as environmental impacts may be thought to be covered by the plenty of environmental impact assessments of agricultural activities itself.
The impression of a ‘blind eye’ on the environment in agricultural RIA may change when publications beyond Web of Science TM Core Collection are considered ( 53 ) or sources other than peer-reviewed journal articles are analysed (e.g. reports; conference proceedings). See, for example, Kelley, et al. ( 38 ), Maredia and Pingali ( 54 ), or FAO ( 55 ). Additionally, scientific publications of the highest quality standard (indicated by reviews and articles being listed in the Web of Science TM Core Collection) seem to not yet reflect experiences and advancements from assessment applications on research and innovation policy that usually include the environmental impact ( 56 ).
Since their beginnings, RIAs have begun to move away from narrow exercises concerned with economic impacts ( 11 ) and expanded their scope to social impacts. However, we only found one sustainability approach in our review that would cover all three impact areas of agricultural research (see ( 57 )). In contrast, progressive approaches to policy impact assessment largely attempt to cover the full range of environmental, social, and economic impacts of policy ( 33 , 58 ). RIAs may learn from them.
Additionally, the focus of agricultural research on technological innovation seems evident. Although the word innovation is sometimes still used for new technology (as in ‘diffusion of innovations’), it is increasingly used for the process of technical and institutional change at the farm level and higher levels of impact. Technology production increasingly is embedded in innovation systems ( 59 ).
The review revealed a diversity of methods (see Table 2 ) applied in impact assessments of agricultural research. In the early phases of RIA, the methods drawn from agricultural economics were considered as good standard for an impact assessment of international agricultural research ( 39 ). However, quantitative methods most often address economic impacts. In addition, the reliability of assessments based on econometric models is often disputed because of strong relationships between modelling assumptions and respective results.
Regarding environmental (or sustainability) impacts of agricultural research, the portfolio of assessment methods could be extended by learning from RIAs in other impact areas. In our literature sample, only review, framework development (e.g. key barrier typologies, environmental costing, or payments for ecosystem services), life-cycle assessment, and semi-structured interviews were used for environmental impacts of agricultural research.
In total, 42 of the 171 analysed papers assessed the impact of participatory research. A co-management of public research acknowledges the influence of the surrounding ecological, social, and political system and allows different types of stakeholder knowledge to shape innovation ( 60 ). Schut, et al. ( 36 ) conceptualize an agricultural innovation support system, which considers multi-stakeholder dynamics next to multilevel interactions within the agricultural system and multiple dimensions of the agricultural problem. Another type of participation in RIAs is the involvement of stakeholders to the evaluation process. A comparatively low number of six papers considered participatory evaluation of research impact, of them three in combination with impact assessment of participatory research.
Approximately 22% of the articles in our sample on agricultural research reported that they conducted their assessments ex ante , but most studies were ex post assessments. Watts, et al. ( 39 ) considered ex ante impact assessment to be more instructive than ex post assessment because it can directly guide the design of research towards maximizing beneficial impacts. This is particularly true when an ex ante assessment is conducted as a comparative assessment comprising a set of alternative options ( 61 ).
Many authors of the studies analysed were not explicit about the time frames considered in their ex post studies. The potential latency of impacts from research points to the need for ex post (and ex ante) studies to account for and analyse longer time periods, either considering ‘decades’ ( 62 , 63 ) or a lag distribution covering up to 50 years, with a peak approximately in the middle of the impact period ( 64 ). This finding is in line with the perspective of impact assessments as an ongoing process throughout a project’s life cycle and not as a one-off process at the end ( 51 ). Nevertheless, ex post assessments are an important component of a comprehensive evaluation package, which includes ex ante impact assessment, impact pathway analysis, programme peer reviews, performance monitoring and evaluation, and process evaluations, among others ( 38 ).
RIA is conceptually and methodologically not yet sufficiently equipped to capture wider societal implications, though ( 14 ). This is due to the specific challenges associated with RIA, including inter alia unknown time lags between research processes and their impacts ( 15–17 ). Independent from their orientation, RIAs are likely to influence research policies for years to come ( 18 ).
However, in the cases in which a RIA is carried out, an increase in the positive impacts (or avoidance of negative impacts) of agricultural research does not follow automatically. Lilja and Dixon ( 65 ) state the following methodological reasons for the missing impact of impact studies: no accountability with internal learning, no developed scaling out, the overlap of monitoring and evaluation and impact assessment, the intrinsic nature of functional and empowering farmer participation, the persistent lack of widespread attention to gender, and the operational and political complexity of multi-stakeholder impact assessment. In contrast, a desired impact of research could be reached or boosted by specific measures without making an impact assessment at all. Kristjanson, et al. ( 66 ), for example, proposed seven framework conditions for agricultural research to bridge the gap between scientific knowledge and action towards sustainable development. RIA should develop into process-oriented evaluations, in contrast to outcome-oriented evaluation ( 67 ), for addressing the intended kind of impacts, the scope of assessment, and for choosing the appropriate assessment method ( 19 ).
This review aimed at providing an overview of impact assessment activities reported in academic agricultural literature with regard to their coverage of impact areas and type of assessment method used. We found a remarkable body of non-scientometric RIA at all evaluation levels of agricultural research but a major interest in economic impacts of new agricultural technologies. These are closely followed by an interest in social impacts at multiple assessments levels that usually focus on food security and poverty reduction and rely slightly more on qualitative assessment methods. In contrast, the assessment of the environmental impacts of agricultural research or comprehensive sustainability assessments was exceptionally limited. They may have been systematically overlooked in the past, for the reason of expected negative results, thought to be covered by other impact studies or methodological challenges. RIA could learn from user-oriented policy impact assessments that usually include environmental impacts. Frameworks for RIA should avoid narrowing the assessment focus and instead considering intended and unintended impacts in several impact areas equally. It seems fruitful to invest in assessment teams’ environmental analytic skills and to expand several of the already developed methods for economic or social impact to the environmental impacts. Only then, the complex and comprehensive contribution of agricultural research to sustainable development can be revealed.
The authors would like to thank Jana Rumler and Claus Dalchow for their support in the Web of Science analysis and Melanie Gutschker for her support in the quantitative literature analysis.
This work was supported by the project LIAISE (Linking Impact Assessment to Sustainability Expertise, www.liaisenoe.eu ), which was funded by Framework Programme 7 of the European Commission and co-funded by the Leibniz-Centre for Agricultural Landscape Research. The research was further inspired and supported by funding from the ‘Guidelines for Sustainability Management’ project for non-university research institutes in Germany (‘Leitfaden Nachhaltigkeitsmanagement’, BMBF grant 311 number 13NKE003A).
Seidl R. et al. ( 2013 ) ‘ Science with Society in the Anthropocene ’, Ambio , 42 / 1 : 5 – 12 .
Google Scholar
OECD . ( 2010 ) ‘Performance-Based Funding for Public Research in Tertiary Education Institutions’, Workshop Proceedings ' 2010. Paris : Organisation for Economic Co-operation and Development .
Hicks D. ( 2012 ) ‘ Performance-based University Research Funding Systems ’, Research Policy , 41 / 2 : 251 – 61 .
Martin B. R. ( 1996 ) ‘ The Use of Multiple Indicators in the Assessment of Basic Research ’, Scientometrics , 36 / 3 : 343 – 62 .
Moed H. F. , Halevi G. ( 2015 ) ‘ Multidimensional Assessment of Scholarly Research Impact ’, Journal of the Association for Information Science and Technology , 66 : 1988 – 2002 .
Penfield T. et al. ( 2014 ) ‘ Assessment, Evaluations, and Definitions of Research Impact: A Review ’, Research Evaluation , 23 / 1 : 21 – 32 .
Meyer R. ( 2011 ) ‘ The Public Values Failures of Climate Science in the US ’, Minerva , 49 / 1 : 47 – 70 .
Bozeman B. , Sarewitz D. ( 2011 ) ‘ Public Value Mapping and Science Policy Evaluation ’, Minerva , 49 / 1 : 1 – 23 .
Helming K. et al. ( 2016 ) ‘ Forschen für nachhaltige Entwicklung. Kriterien für gesellschaftlich verantwortliche Forschungsprozesse (Research for Sustainable Development. Criteria for Socially Responsible Research Processes) ’, GAIA , 25 / 3 : 161 – 5 .
Cagnin C. , Amanatidou E. , Keenan M. ( 2012 ) ‘ Orienting European Innovation Systems Towards Grand Challenges and the Roles that FTA Can Play ’, Science and Public Policy , 39 / 2 : 140 – 52 .
Godin B. , Doré C. ( 2004 ) Measuring the Impacts of Science: Beyond the Economic Dimension . Montréal (Québec) : Centre Urbanisation Culture Société (INRS) .
Ferretti J. et al. ( 2016 ) Reflexionsrahmen für Forschen in gesellschaftlicher Verantwortung. (Framework for Reflecting Research in Societal Responsibility) . Berlin : Federal Ministry of Education and Research (BMBF) .
Jacobsson S. , Vico E. P. , Hellsmark H. ( 2014 ) ‘ The Many Ways of Academic Researchers: How is Science Made Useful? ’, Science and Public Policy , 41 : 641 – 57 .
Levitt R. et al. ( 2010 ) Assessing the Impact of Arts and Humanities Research at the University of Cambridge . Cambridge : University of Cambridge .
Donovan C. ( 2011 ) ‘ State of the Art in Assessing Research Impact: Introduction to a Special Issue ’, Research Evaluation , 20 / 3 : 175 – 9 .
Ekboir J. ( 2003 ) ‘ Why Impact Analysis Should not be Used for Research Evaluation and what the Alternatives Are ’, Agricultural Systems , 78 / 2 : 166 – 84 .
Morton S. ( 2015 ) ‘ Progressing Research Impact Assessment: A ‘Contributions’ Approach ’, Research Evaluation , 24 : 405 – 19 .
Reinhardt A. ( 2013 ) ‘Different Pathways to Impact? “Impact” and Research Fund Allocation in Selected European Countries’, in Dean A. , Wykes M. , Stevens H. (eds) 7 Essays on Impact. DESCRIBE Project Report for Jisc , pp. 88 – 101 . Exeter : University of Exeter .
Google Preview
European Science Foundation . ( 2012 ) The Challenges of Impact Assessment. Working Group 2: Impact Assessment . Strasbourg : European Science Foundation .
Guthrie S. et al. ( 2013 ) Measuring Research. A Guide to Research Evaluation Frameworks and Tools . Cambridge : RAND Corporation .
Alston J. M. et al. ( 2011 ) ‘ The Economic Returns to US Public Agricultural Research ’, American Journal of Agricultural Economics , 93 / 5 : 1257 – 77 .
Spaapen J. , Drooge L. ( 2011 ) ‘ Introducing' Productive Interactions' in Social Impact Assessment ’, Research Evaluation , 20 / 3 : 211 – 18 .
Bozeman B. ( 2003 ) Public Value Mapping of Science Outcomes: Theory and Method . Washington : Center for Science, Policy and Outcomes .
Milat A. J. , Bauman A. E. , Redman S. ( 2015 ) ‘ A Narrative Review of Research Impact Assessment Models and Methods ’, Health Research Policy and Systems , 13 / 1 : 18.
Bell S. , Shaw B. , Boaz A. ( 2011 ) ‘ Real-world Approaches to Assessing the Impact of Environmental Research on Policy ’, Research Evaluation , 20 / 3 : 227 – 37 .
Gaunand A. et al. ( 2015 ) ‘ How Does Public Agricultural Research Impact Society? A Characterization of Various Patterns ’, Research Policy , 44 / 4 : 849 – 61 .
Bokelmann W. et al. ( 2012 ) Sector Study on the Analysis of the Innovation of German Agriculture (Sektorstudie zur Untersuchung des Innovationssystems der deutschen Landwirtschaft) . Berlin : Federal Office for Agriculture and Food (BLE) .
Weißhuhn P. , Helming K. ( 2015 ) ‘Methods for Assessing the Non-Scientometric Impacts of Agricultural Research: A Review’. In ImpAR Conference 2015: Impacts of Agricultural Research-Towards an Approach of Societal V alues. Paris: INRA.
European Science Foundation . ( 2009 ) Evaluation in National Research Funding Agencies: Approaches, Experiences and Case Studies . Strasbourg : European Science Foundation .
Bozeman B. ( 2000 ) ‘ Technology Transfer and Public Policy: A Review of Research and Theory ’, Research Policy , 29 / 4 : 627 – 55 .
Hummer K. E. , Hancock J. F. ( 2015 ) ‘ Vavilovian Centers of Plant Diversity: Implications and Impacts ’, Hortscience , 50 / 6 : 780 – 3 .
EC . ( 2015 ) Better Regulation “Toolbox” . Brussels : European Commission .
Helming K. et al. ( 2013 ) ‘ Mainstreaming Ecosystem Services in European Policy Impact Assessment ’, Ecosystem Services in EIA and SEA , 40 : 82 – 7 .
Thapa D. B. et al. ( 2009 ) ‘ Identifying Superior Wheat Cultivars in Participatory Research on Resource Poor Farms ’, Field Crops Research , 112 / 2–3 : 124 – 30 .
Holdsworth M. et al. ( 2015 ) ‘ African Stakeholders' Views of Research Options to Improve Nutritional Status in Sub-Saharan Africa ’, Health Policy and Planning , 30 / 7 : 863 – 74 .
Schut M. et al. ( 2015 ) ‘ RAAIS: Rapid Appraisal of Agricultural Innovation Systems (Part I). A Diagnostic Tool for Integrated Analysis of Complex Problems and Innovation Capacity ’, Agricultural Systems , 132 : 1 – 11 .
Jones M. M. , Grant J. ( 2013 ) ’Making the Grade: Methodologies for assessing and evidencing research impact’. In Dean A. , Wykes M. , Stevens H. (eds) 7 Essays on Impact. DESCRIBE Project Report for Jisc , pp. 25 – 43 . Exeter : University of Exeter .
Kelley T. , Ryan J. , Gregersen H. ( 2008 ) ‘ Enhancing Ex Post Impact Assessment of Agricultural Research: The CGIAR Experience ’, Research Evaluation , 17 / 3 : 201 – 12 .
Watts J. et al. ( 2008 ) ‘ Transforming Impact Assessment: Beginning the Quiet Revolution of Institutional Learning and Change ’, Experimental Agriculture , 44 / 1 : 21 – 35 .
Bennett J. W. , Kelley T. G. , Maredia M. K. ( 2012 ) ‘ Integration of Environmental Impacts Into Ex-post Assessments of International Agricultural Research: Conceptual Issues, Applications, and the Way Forward ’, Research Evaluation , 21 / 3 : 216 – 28 .
Maredia M. K. , Raitzer D. A. ( 2012 ) ‘ Review and Analysis of Documented Patterns of Agricultural Research Impacts in Southeast Asia ’, Agricultural Systems , 106 / 1 : 46 – 58 .
Renkow M. , Byerlee D. ( 2010 ) ‘ The Impacts of CGIAR Research: A Review of Recent Evidence ’, Food Policy , 35 / 5 : 391 – 402 .
Raitzer D. A. , Maredia M. K. ( 2012 ) ‘ Analysis of Agricultural Research Investment Priorities for Sustainable Poverty Reduction in Southeast Asia ’, Food Policy , 37 / 4 : 412 – 26 .
Ajayi O. C. et al. ( 2011 ) ‘ Agricultural Success from Africa: The Case of Fertilizer Tree Systems in Southern Africa (Malawi, Tanzania, Mozambique, Zambia and Zimbabwe) ’, International Journal of Agricultural Sustainability , 9 / 1 : 129 – 36 .
Cavallo E. et al. ( 2014 ) ‘ Strategic Management Implications for the Adoption of Technological Innovations in Agricultural Tractor: The Role of Scale Factors and Environmental Attitude ’, Technology Analysis and Strategic Management , 26 / 7 : 765 – 79 .
Briones R. M. et al. ( 2008 ) ‘ Priority Setting for Research on Aquatic Resources: An Application of Modified Economic Surplus Analysis to Natural Resource Systems ’, Agricultural Economics , 39 / 2 : 231 – 43 .
Kyllmar K. et al. ( 2014 ) ‘ Small Agricultural Monitoring Catchments in Sweden Representing Environmental Impact ’, Agriculture, Ecosystems and Environment , 198 : 25 – 35 .
Skinner J. et al. ( 1997 ) ‘ An Overview of the Environmental Impact of Agriculture in the UK ’, Journal of Environmental Management , 50 / 2 : 111 – 28 .
Van der Werf H. M. , Petit J. ( 2002 ) ‘ Evaluation of the Environmental Impact of Agriculture at the Farm Level: A Comparison and Analysis of 12 Indicator-based Methods ’, Agriculture, Ecosystems and Environment , 93 / 1 : 131 – 45 .
Carruthers G. , Vanclay F. ( 2012 ) ‘ The Intrinsic Features of Environmental Management Systems that Facilitate Adoption and Encourage Innovation in Primary Industries ’, Journal of Environmental Management , 110 : 125 – 34 .
Morris M. et al. ( 2003 ) ‘ Assessing the Impact of Agricultural Research: An Overview ’, Quarterly Journal of International Agriculture , 42 / 2 : 127 – 48 .
Podhora A. et al. ( 2013 ) ‘ The Policy-Relevancy of Impact Assessment Tools: Evaluating Nine Years of European Research Funding ’, Environmental Science and Policy , 31 : 85 – 95 .
Rodrigues G. S. , de Almeida Buschinelli C. C. , Dias Avila A. F. ( 2010 ) ‘ An Environmental Impact Assessment System for Agricultural Research and Development II: Institutional Learning Experience at Embrapa ’, Journal of Technology Management and Innovation , 5 / 4 : 38 – 56 .
Maredia M. , Pingali P. ( 2001 ) Environmental Impacts of Productivity-Enhancing Crop Research: A Critical Review . Durban : CGIAR .
FAO . ( 2011 ) ‘ Environmental Impact Assessment', Guideline for FAO field projects . Rome : Food and Agriculture Organization of the United Nations .
Miedzinski M. et al. ( 2013 ) Assessing Environmental Impacts of Research and Innovation Policy .
Ervin D. E. , Glenna L. L. , Jussaume R. A. ( 2011 ) ‘ The Theory and Practice of Genetically Engineered Crops and Agricultural Sustainability ’, Sustainability , 3 / 6 : 847 – 74 .
Jacob K. et al. ( 2012 ) ‘Sustainability in Impact Assessments - A Review of Impact Assessment Systems in selected OECD countries and the European Commission’ . Paris : Organisation for Economic Co-operation and Development .
Röling N. ( 2009 ) ‘ Pathways for Impact: Scientists' Different Perspectives on Agricultural Innovation ’, International Journal of Agricultural Sustainability , 7 / 2 : 83 – 94 .
Dentoni D. , Klerkx L. ( 2015 ) ‘ Co-managing Public Research in Australian Fisheries Through Convergence-Divergence Processes ’, Marine Policy , 60 : 259 – 71 .
Helming K. et al. ( 2011 ) ‘ Ex Ante Impact Assessment of Policies Affecting Land Use, Part A: Analytical Framework ’, Ecology and Society , 16 / 1 : 27 .
Stads G. J. , Beintema N. ( 2015 ) ‘ Agricultural R&D Expenditure in Africa: An Analysis of Growth and Volatility ’, European Journal of Development Research , 27 / 3 : 391 – 406 .
Raitzer D. A. , Kelley T. G. ( 2008 ) ‘ Benefit-cost Meta-analysis of Investment in the International Agricultural Research Centers of the CGIAR ’, Agricultural Systems , 96 / 1-3 : 108 – 23 .
Andersen M. A. ( 2015 ) ‘ Public Investment in US Agricultural R&D and the Economic Benefits ’, Food Policy , 51 : 38 – 43 .
Lilja N. , Dixon J. ( 2008 ) ‘ Responding to the Challenges of Impact Assessment of Participatory Research and Gender Analysis ’, Experimental Agriculture , 44 / 1 : 3 – 19 .
Kristjanson P. et al. ( 2009 ) ‘ Linking International Agricultural Research Knowledge with Action for Sustainable Development ’, Proceedings of the National Academy of Sciences United States of America , 106 / 13 : 5047 – 52 .
Upton S. , Vallance P. , Goddard J. ( 2014 ) ‘ From Outcomes to Process: Evidence for a New Approach to Research Impact Assessment ’, Research Evaluation , 23 : 352 – 65 .
The exact TOPIC query was: agricult* NEAR/1 (research* OR *scien* OR "R&D" OR innovati*) AND (research* OR *scien* OR "R&D" OR innovati*) NEAR/2 (impact* OR assess* OR evaluat* OR criteria* OR benefit* OR adoption* OR adaptation*)
The exact TITLE query was: agricult* AND (research* OR *scien* OR "R&D" OR innovati*) AND (impact* OR assess* OR evaluat* OR criteria* OR benefit* OR adoption* OR adaptation*)
Month: | Total Views: |
---|---|
October 2017 | 65 |
November 2017 | 87 |
December 2017 | 227 |
January 2018 | 565 |
February 2018 | 472 |
March 2018 | 649 |
April 2018 | 539 |
May 2018 | 456 |
June 2018 | 409 |
July 2018 | 335 |
August 2018 | 544 |
September 2018 | 502 |
October 2018 | 494 |
November 2018 | 559 |
December 2018 | 468 |
January 2019 | 384 |
February 2019 | 469 |
March 2019 | 407 |
April 2019 | 296 |
May 2019 | 290 |
June 2019 | 297 |
July 2019 | 304 |
August 2019 | 264 |
September 2019 | 300 |
October 2019 | 381 |
November 2019 | 434 |
December 2019 | 339 |
January 2020 | 342 |
February 2020 | 343 |
March 2020 | 282 |
April 2020 | 154 |
May 2020 | 160 |
June 2020 | 161 |
July 2020 | 137 |
August 2020 | 142 |
September 2020 | 363 |
October 2020 | 527 |
November 2020 | 278 |
December 2020 | 175 |
January 2021 | 214 |
February 2021 | 240 |
March 2021 | 296 |
April 2021 | 177 |
May 2021 | 207 |
June 2021 | 233 |
July 2021 | 158 |
August 2021 | 169 |
September 2021 | 360 |
October 2021 | 328 |
November 2021 | 294 |
December 2021 | 236 |
January 2022 | 224 |
February 2022 | 274 |
March 2022 | 294 |
April 2022 | 156 |
May 2022 | 173 |
June 2022 | 173 |
July 2022 | 163 |
August 2022 | 136 |
September 2022 | 250 |
October 2022 | 277 |
November 2022 | 207 |
December 2022 | 156 |
January 2023 | 203 |
February 2023 | 240 |
March 2023 | 257 |
April 2023 | 210 |
May 2023 | 226 |
June 2023 | 176 |
July 2023 | 207 |
August 2023 | 175 |
September 2023 | 318 |
October 2023 | 260 |
November 2023 | 242 |
December 2023 | 138 |
January 2024 | 275 |
February 2024 | 242 |
March 2024 | 314 |
April 2024 | 218 |
May 2024 | 187 |
June 2024 | 135 |
July 2024 | 151 |
August 2024 | 236 |
September 2024 | 129 |
Email alerts
Citing articles via.
- Recommend to your Library
Affiliations
- Online ISSN 1471-5449
- Print ISSN 0958-2029
- Copyright © 2024 Oxford University Press
- About Oxford Academic
- Publish journals with us
- University press partners
- What we publish
- New features
- Open access
- Institutional account management
- Rights and permissions
- Get help with access
- Accessibility
- Advertising
- Media enquiries
- Oxford University Press
- Oxford Languages
- University of Oxford
Oxford University Press is a department of the University of Oxford. It furthers the University's objective of excellence in research, scholarship, and education by publishing worldwide
- Copyright © 2024 Oxford University Press
- Cookie settings
- Cookie policy
- Privacy policy
- Legal notice
This Feature Is Available To Subscribers Only
Sign In or Create an Account
This PDF is available to Subscribers Only
For full access to this pdf, sign in to an existing account, or purchase an annual subscription.

An official website of the United States government
Official websites use .gov A .gov website belongs to an official government organization in the United States.
Secure .gov websites use HTTPS A lock ( Lock A locked padlock ) or https:// means you’ve safely connected to the .gov website. Share sensitive information only on official, secure websites.
Research and Science
From fostering continued economic growth to adapting to the effects of climate change and addressing food security, the United States can continue to be a leader in global agriculture. Each day, the work of USDA scientists and researchers touches the lives of all Americans - from the farm field to the kitchen table and from the air we breathe to the energy that powers our country.
The challenges facing agriculture, natural resources, and conservation are immense and can be addressed through robust research enterprise and educational programs. USDA intramural and extramural science helps to protect, secure, and improve our food, agricultural and natural resources systems.
USDA Science and Research Strategy, 2023-2026: Cultivating Scientific Innovation
The “ USDA Science and Research Strategy, 2023-2026: Cultivating Scientific Innovation (PDF, 21.4 MB)” presents a near-term vision for transforming U.S. agriculture through science and innovation, and outlines USDA’s highest scientific priorities. The S&RS is a call to action for USDA partners, stakeholders, and customers to join the conversation and help identify innovative research strategies that lead to real-world, practical solutions that help farmers, producers, and communities thrive.
Learn more and engage below:
USDA Science and Research Strategy
AGARDA: A Vision for Disruptive Science to Confront Audacious Challenges
Agriculture Advanced Research and Development Authority (AGARDA) Implementation Strategy (PDF, 1.8 MB) is a framework outlining a new approach for delivering disruptive breakthrough discoveries for agriculture.
Strengthening Our Research System
USDA has refocused its science agencies to ensure the most effective and efficient use of its resources, while leveraging the strengths of our partners across the scientific community.
The Office of the Chief Scientist (OCS) coordinates USDA research, education and Extension with scientists and researchers across the federal government and university and private partners, to make the best use of taxpayer investments. In 2012, OCS continued focus on the Research, Education and Economics Action Plan (PDF, 486 KB) and identified seven priority research topics:
- Global Food Supply and Security
- Climate and Energy Needs
- Sustainable Use of Natural Resources
- Nutrition and Childhood Obesity
- Food Safety
- Education and Science Literacy
- Rural-urban Interdependence/Rural Prosperity
The Agricultural Research Service (ARS) conducts research to develop and transfer solutions to agricultural problems of high national priority.
The Economic Research Service (ERS) , through science-based economic research and analysis, informs public policy and other decisions about agriculture, food, rural development, and environmental challenges.
The National Agricultural Statistics Service (NASS) conducts hundreds of surveys every year and prepares reports covering virtually every aspect of U.S. agriculture.
The National Institute of Food and Agriculture (NIFA) supports research, education and Extension programs in the Land-Grant University System and other partner organizations.
Enhancing the Productivity of American Agriculture and Ensuring the Safety of our Food Supply
USDA invests in research, development, and outreach of new varieties and technologies to mitigate animal/plant diseases and increase productivity, sustainability, and product quality. USDA research has supported America's farmers and ranchers in their work to produce a safe and abundant food supply for over 100 years. This work has helped feed the nation and sustain an agricultural trade surplus since the 1960s.
An additional focus is to establish more sustainable systems that enhance crop and animal health. Our scientists and university partners have revealed the genetic blueprints of a host of plants and animals including the genomes of apples, pigs, and turkeys, and in 2012, they furthered understanding of the tomato, bean, wheat and barley genomes -- key drivers in developing the resilience of those crops to feed growing populations.
NASS has developed animated U.S. crop progress and topsoil moisture maps , along with other resources, to help experts assess farmland data. USDA researchers also created the Maize Genome Database, an important tool to help farmers improve traits in a crop vital to the world. Meeting growing global demand for food, fiber, and biofuel requires robust investment in agricultural research and development (R&D) from both public and private sectors. USDA is a leader in remote sensing and mapping to visualize data in support of agricultural policy and business decision making as well as program operation. We ranked first worldwide among research institutions publishing on priority diseases in animal health including salmonellosis, avian influenza , mycobacterial disease, coccidiosis, campylobacterosis, mastitis and others.
USDA conducts and supports science that informs decisions and policies contributing to a safe food supply and the reduction of foodborne hazards. Our scientists found the primary site where the virus that causes foot-and-mouth disease begins infection in cattle and developed an improved vaccine against the disease. They are also working on new strategies to control mites and other major honey bee problems such as colony collapse disorder .
Improving Nutrition and Confronting Obesity
USDA builds the evidence base for food-based and physical activity strategies and develops effective education activities to promote health and reduce malnutrition and obesity in children and high-risk populations. For example, ARS evaluated school characteristics associated with healthier or less healthy food preparation practices and offerings and found that the school nutrition environment could be improved by requiring food service managers to hold nutrition-related college degrees, pass a food service training program, and by participating in a school-based nutrition program such as USDA Team Nutrition .
USDA-supported science is investigating the causes of childhood obesity so that our country can address the epidemic. In these efforts, USDA supports nutrition education programs and encourages Americans to consume more nutritious foods like fruits and vegetables. Our scientists are part of an international team that has found a way to boost the nutritional value of broccoli, tomatoes and corn, and have worked to find ways to bolster the nutritional content of other staple crops like oats and rice. USDA research has supported these efforts, showing how healthy foods can often cost less than foods that are high in saturated fat, added sugar and/or sodium.
In 2013, USDA updated the national assessment of urban and rural food deserts - low-income areas with limited access to affordable and nutritious food - and provided information on the socioeconomic and demographic characteristics that distinguish food deserts from other areas, for decision-makers and stakeholders concerned about access to healthy foods.
Conserving Natural Resources and Combating Climate Change
USDA develops and delivers science-based knowledge that empowers farmers, foresters, ranchers, landowners, resource managers, policymakers, and Federal agencies to manage the risks, challenges, and opportunities of climate variability, and that informs decision-making and improves practices in environmental conservation.
Our scientists are developing rice and corn crops that are drought- and flood-resistant and helping to improve the productivity of soil, as well as production systems that require increasing smaller amounts of pesticides or none at all.
Vegetation indices contained in VegScape have proven useful for assessing crop condition and identifying the aerial extent of floods, drought, major weather anomalies, and vulnerabilities of early/late season crops. This tool allows users to monitor and track weather anomalies' effects on crops in near real time and compare this information to historical data on localized levels or across States.
Additionally, our researchers have examined the potential impacts of a suite of climate scenarios on U.S. crop production. Studies like these will help policymakers, farmers, industry leaders and others better understand and adapt to a changing climate on America's crop production.
Our researchers created i-Tree , urban forest management software to help cities understand the value of urban trees through carbon sequestration, erosion protection, energy conservation and water filtration, and since 2009 have continued building on the success of the tool and expanding its use. Our scientists are conducting research on uses of wood, helping companies meet green building design standards and creating jobs using forest products. We have also worked with Major League Baseball to reduce the occurrence of broken baseball bats.
USDA supports families managing through tough economic times by helping residents save energy at home and conserve water, with a program run by Cooperative Extension and our land-grant university partners. Cooperative Extension-affiliated volunteer monitoring programs have engaged citizens in water monitoring to better understand the effects of climate change and/or aquatic invasive species on local waters. Collectively, these programs interacted with hundreds of local, State, and Federal partners. The programs help citizens detect the presence of invasive species and harmful algal blooms.
Science Education and Extension
USDA recognizes the importance of recruiting, cultivating, and developing the next generation of scientists, leaders, and a highly skilled workforce for food, agriculture, natural resources, forestry, environmental systems, and life sciences.
The NIFA interagency agreement with the U.S. Fish and Wildlife Service leverages technology and innovation and involves youth in STEM outreach and exposure. Youth participants developed science process skills related to using GIS and research design, analyzing and interpreting data, and reporting findings to the community which has enabled them to become better consumers of science and citizens capable of making wise STEM policy choices.
USDA strives to provide effective research, education, and extension activities that inform public and private decision-making in support of rural and community development . NASS holds outreach events throughout the Census cycle with underserved and minority and disadvantaged farming groups to promote participation in the Census of Agriculture . With funding and support from NIFA, many Tribal Colleges are offering Reservation citizens training ranging from basic financial literacy to business start-up and marketing information so that families not only survive, but thrive.
In addition, the ERS Atlas of Rural and Small Town America brings together over 80 demographic, economic, and agricultural statistics for every county in all 50 states and assembles statistics in four broad categories -- people, jobs, agriculture, and geography.
Research and Science Centers and Databases
- Agricultural Network Information Center (AGNIC)
- Agricultural Online Access (AGRICOLA)
- Alternative Farming Systems Information Center (AFSIC)
- Animal Welfare Information Center (AWIC)
- Current Research Information Center (CRIS)
- Digital Desktop (DigiTop) for Employees
- Food and Nutrition Assistance Research Database
- Food and Nutrition Information Center
- Production, Supply and Distribution Online (PSD Online) Database
- Rural Information Center
- Water and Agricultural Information Center
Thank you for visiting nature.com. You are using a browser version with limited support for CSS. To obtain the best experience, we recommend you use a more up to date browser (or turn off compatibility mode in Internet Explorer). In the meantime, to ensure continued support, we are displaying the site without styles and JavaScript.
- View all journals
- Explore content
- About the journal
- Publish with us
- Sign up for alerts
- Open access
- Published: 09 September 2021
Advancing agricultural research using machine learning algorithms
- Spyridon Mourtzinis 1 ,
- Paul D. Esker 2 ,
- James E. Specht 3 &
- Shawn P. Conley 4
Scientific Reports volume 11 , Article number: 17879 ( 2021 ) Cite this article
10k Accesses
14 Citations
34 Altmetric
Metrics details
- Agroecology
Rising global population and climate change realities dictate that agricultural productivity must be accelerated. Results from current traditional research approaches are difficult to extrapolate to all possible fields because they are dependent on specific soil types, weather conditions, and background management combinations that are not applicable nor translatable to all farms. A method that accurately evaluates the effectiveness of infinite cropping system interactions (involving multiple management practices) to increase maize and soybean yield across the US does not exist. Here, we utilize extensive databases and artificial intelligence algorithms and show that complex interactions, which cannot be evaluated in replicated trials, are associated with large crop yield variability and thus, potential for substantial yield increases. Our approach can accelerate agricultural research, identify sustainable practices, and help overcome future food demands.
Similar content being viewed by others
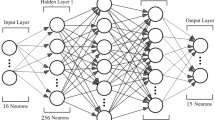
Simultaneous multi-crop land suitability prediction from remote sensing data using semi-supervised learning
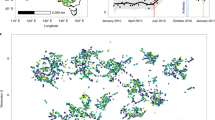
Explainable machine learning models of major crop traits from satellite-monitored continent-wide field trial data
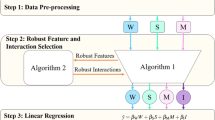
An interaction regression model for crop yield prediction
Introduction.
Increasing food demand will challenge the agricultural sector globally over the next decades 1 . A sustainable solution to this challenge is to increase crop yield without massive cropland area expansion. This can be achieved by identifying and adopting best management practices. To do so requires a more detailed understanding of how crop yield is impacted by climate change 2 , 3 and growing-season weather variability 4 . Even with that knowledge, prediction is challenging because various factors interact with each other. For example, variability in soil type can interact with weather conditions and mitigate or aggravate climate-related impacts on crop yield 5 , 6 . Additionally, seed genetics (G) and crop management decisions (M), interact with the effect of environment (E: soil and in-season weather conditions), thereby resulting in a near infinite number of combinations of G × E × M that can impact crop yield.
Substantial variability in crop yield arises from the wide range of optimal to sub-optimal management observed in soybean farmers’ fields 7 , 8 . Reducing the frequency of lowest vs . highest yields has been proposed as an effective means to increase food production in existing crop land 9 . In that regard, replicated field experiments have been used to identify best management practices for several decades. Most commonly, the effectiveness of up to three management factors and their interactions are evaluated in a single location due to practical constraints (e.g., cost, logistics). By holding the background management constant, causal relationships are identified, and the effectiveness of the examined management practice/s is assessed. It is assumed that background management practices are optimal or at least relevant to what most farmers use in the region, which in fact may not be realistic for many farmers.
Multi-year-site performance trials that account for large environmental and background management variability is another common practice in agricultural research. Such trials usually estimate an average effect across environments and background cropping systems. Inevitably, the measured yield response magnitude and sign may not apply to all farms in the examined region. Other research approaches involve analysis of producer self-reported data 7 , 8 , which can capture yield trends attributable to producer management choice across large regions, but such studies lack sufficient power relative to establishing causality and evaluating complex high-order G × E × M interactions.
Process-based models have been extensively used to evaluate the effect of weather 10 and management 11 , 12 on crop yield. However, to obtain accurate estimates, the models require extensive calibration, which is not a trivial task due to the large number of parameters. Specifically, it has been shown that management is an important source of uncertainty in process-based models, which can lead to substantial and varying degree of bias in yield estimates across the US, even when using harmonized parameters 13 .
Given all the well-known deficiencies of current agricultural research methods, we argue that a method that allows environment-specific identification of unique cropping systems with the greatest yield potential is essential to meet future food demand. Here, by utilizing maize and soybean yield and management data from publicly available performance tests, plus associated weather data, and by leveraging the power of machine learning (ML) algorithms, we developed a method that can evaluate myriads of potential crop management systems and thereby identify those with the greatest yield potential in specific environments across the US.
Results and discussion
Two databases including yield, management, and weather data for maize (n = 17,013) and soybean (n = 24,848) involving US crop performance trials conducted in 28 states between 2016 to 2018 for maize and between 2014 to 2018 for soybean, were developed (Fig. 1 ). Crop yield and management data were obtained from publicly available variety performance trials which are typically performed yearly in several locations across each state ( see methods for more information ). Final databases were separated in training (80% of database) and testing (20% of database) datasets using stratified sampling by year, use of irrigation, and soil type. For each crop, an extreme gradient boosting (XGBoost, see methods for more information ) algorithm to estimate yield based on soil type and weather conditions (E), seed traits (G) and management practices (M) was developed (see variables listed in Tables S1 and S2 for maize and soybean, respectively, and data science workflow in Fig. S1 ).
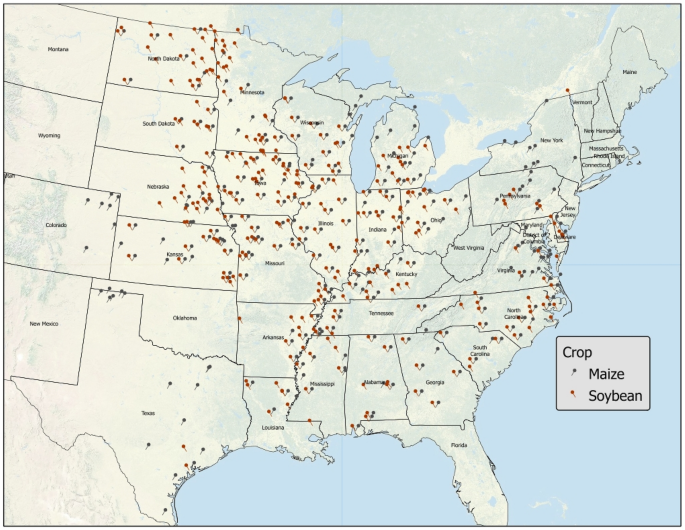
Locations where maize and soybean trials were performed during the examined period. The map was developed in ArcGIS Pro 2.8.0 ( https://www.esri.com ).
The developed algorithms exhibited a high degree of accuracy when estimating yield in independent datasets (test dataset not used for model calibration) (Fig. 2 ). For maize, the root mean square error (RMSE) and mean absolute error (MAE) was a respective 4.7 and 3.6% of the dataset average yield (13,340 kg/ha). For soybean, the respective RMSE and MAE was 6.4 and 4.9% of the dataset average yield (4153 kg/ha). As is evident in the graphs (Fig. 2 ), estimated yields exhibited a high degree of correlation with actual yields for both algorithms in the independent datasets. For maize and soybean, 72.3 and 60% of cases in the test dataset deviated less than 5% from actual yields, respectively. Maximum deviation for maize and soybean reached 43 and 70%, respectively. Data points with deviations greater than 15% from actual yield were 1.5% in maize and 3.6% in soybean databases. These results suggest that the developed algorithms can accurately estimate maize and soybean yields utilizing database-generated information involving reported environmental, seed genetic, and crop management variables.
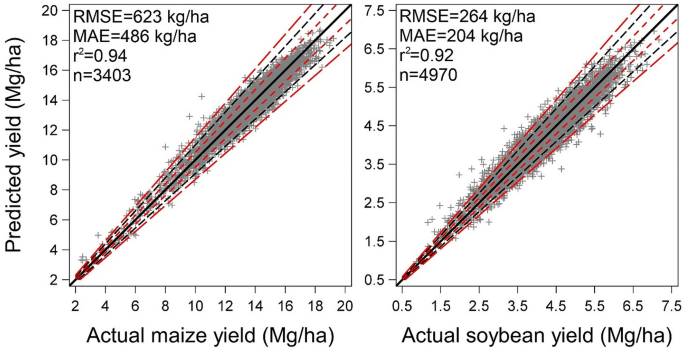
Actual versus algorithm-derived maize (left) and soybean (right) yield in test datasets. Black solid line indicates y = x, red short-dashed lines, black dashed lines, and red long-dashed lines indicate ± 5, 10, and 15% deviation from the y = x line. RMSE, root mean square error; MAE, mean absolute error; r 2 , coefficient of determination; n = number of observations. Each observation corresponds to a yield of an individual cropping system in a specific environment (location-year).
In contrast to statistical models, ML algorithms can be complex, and the effect of single independent variables may not obvious. However, accumulated local effects (ALE) plots 14 can aid the understanding and visualization of important and possibly correlated features in ML algorithms. For both crops, indicatively important variables included sowing date, seeding rate, nitrogen fertilizer (for maize), row spacing (for soybean) and June to September cumulative precipitation (Fig. 3 ). Across the entire region and for both crops, the algorithm-derived trends suggest that above average yields occur in late April to early May sowing dates, but sharply decrease thereafter. Similar responses have been observed in many regional studies across the US for both, maize 15 , 16 , 17 , 18 and soybean 19 . Similarly, simulated yield curves due to increasing seeding rate are in close agreement with previous maize 20 , 21 and soybean 22 studies. The maize algorithm has captured the increasing yield due to increasing N fertilizer rate. The soybean algorithm suggests that narrower row spacing resulted in above average yield compared to wider spacing. Such response has been observed in many regions across the US 23 . Season cumulative precipitation between 400 and 700 mm resulted in above average yields for both crops.
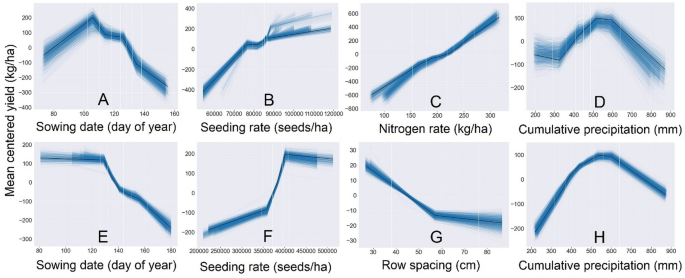
Accumulated local effect plots for maize sowing date ( A ), seeding rate ( B ), Nitrogen fertilizer rate ( C ), and cumulative precipitation between June and September (mm) ( D ), and soybean sowing date ( E ), seeding rate ( F ), row spacing ( G ), and cumulative precipitation between June and September (mm) ( H ).
The responses in the ALE plots (Fig. 3 ) suggest that these algorithms have captured the general expected average responses for important single features. Nevertheless, our databases include hundreds of locations with diverse environments across the US and site-specific crop responses which may vary due to components of the G × E × M interaction. We argue that, instead of examining a single or low-order management interactions, site-specific evaluation of complex high order interactions (a.k.a. cropping systems) can reveal yield differences that current research approaches cannot fully explore and quantify. For example, sowing date exerts a well-known impact on maize and soybean yield. For each crop separately, by creating a hypothetical cropping system (a single combination of all management and traits in Tables S1 and S2 ) in a randomly chosen field in south central Wisconsin (latitude = 43.34, longitude = -89.38), and by applying the developed algorithms, we can generate estimates of maize and soybean yield. For that specific field and cropping system (out of the vast number of management combinations a farmer can choose from), maize yield with May 1st sowing was 711 kg/ha greater (6% increase) than June sowing (Fig. 4 A). By creating scenarios with 256 background cropping system choices (Table S3 ), the resultant algorithm-derived yield estimate difference for the same sowing date contrast (averaged across varying cropping systems) was smaller but still positive (3% increase), although the range of possible yield differences was wider (Fig. 4 B). However, when comparing, instead of averaging, the estimated yield potential among the simulated cropping systems, a 2903 kg/ha yield difference (25% difference) was observed (Fig. 4 C). Interestingly, when focusing on the early sown fields that were expected to exhibit the greatest yield, the same yield difference was observed (Fig. 4 D). This result shows that sub-optimal background management can mitigate the beneficial effect of early sowing (Table S4 ).
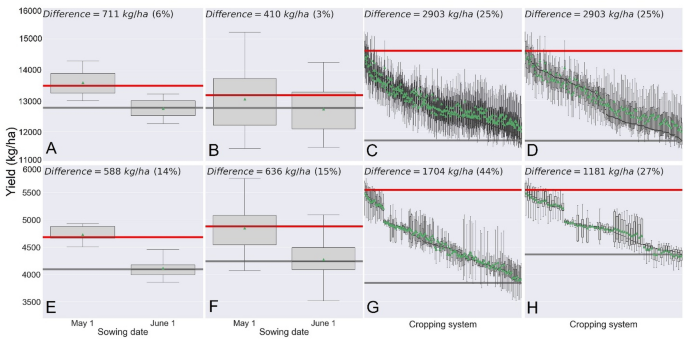
Maize yield difference (in kg/ha and percentage) due to sowing date (May 1st vs. June 1st) for a single identical background cropping system ( A ), maize yield difference due to sowing date when averaged across 256 (3 years × 256 cropping systems = 768 year-specific yields) ( B ), maize yield variability in each of the 256 cropping systems ( C ), and maize yield variability in each of the 128 cropping systems with early sowing ( D ). Soybean yield difference due to sowing date (May 1st vs June 1st) for a single identical background cropping system ( E ), soybean yield difference due to sowing date when averaged across 128 (5 years × 128 cropping systems = 640 year-specific yields) ( F ), soybean yield in each of the 128 cropping systems ( G ) and soybean yield variability due in each of the 64 cropping systems with early sowing ( H ). Within each panel, the horizontal red and grey lines indicate the boxplot with maximum and minimum yield, respectively. In the left four panels, boxes delimit first and third quartiles; solid lines inside boxes indicate median and green triangles indicate means. Upper and lower whiskers extend to maximum and minimum yields. Each maize and soybean cropping system is a respective 8-way and a 7-way interaction of management practices in a randomly chosen field in Wisconsin, USA (Table S3 and S5 , respectively).
In the case of soybean, a May 1st sowing resulted in greater yield (588 kg/ha; a 14% increase) than a June 1st in the single background cropping system (Fig. 4 E). The result was consistent when yield differences due to sowing date were averaged across 128 background cropping system choices (Table S5 ) (Fig. 4 F). Similar to what was observed in maize, among all cropping systems, yield varied by 1704 kg/ha (44% difference) (Fig. 4 G). When focusing only on the early sown fields, a 1181 kg/ha yield difference (27% yield increase) was observed (Fig. 4 H). In agreement with maize, this result highlights the importance of accounting for sub-optimal background management which can mitigate the beneficial effect of early sowing (Table S6 ).
We note here the ability of farmers to change management practices can be limited due to an equipment constraint (e.g., change planter unit row width) or simply impossible (e.g., change the previous year’s crop). Thus, recommended management practices that were evaluated in studies that used specific background management may not be applicable in some instances. The benefits of the foregoing approach, which involves extensive up-to-date agronomic datasets and high-level computational programing, can have important and immediate implications in future agricultural trials. Our approach allows for more precise examination of complex management interactions in specific environments (soil type and growing season weather) across the US (region covered in Fig. 1 ). The ability to extract single management practice information (even across cropping systems) is also possible by utilizing ALE plots, or by calculation of the frequency at which a given level/rate of a management practice appeared among the highest yielding cropping systems (Tables S4 and S6 ).
Among all available 30-d weather variables, many were strongly correlated in both crop databases (Figs. S2 and S3 for maize and soybean, respectively). Models using all 30-d interval variables with r < 0.7 (Tables S8 and S9 ) showed minimal to no performance gain compared to the final more parsimonious models that included season-long weather variables (Fig. S4 ). Thus, we consider the length of periods we chose to represent well the approximate successive 60-d pre-sowing, 120-d in-season, and 60-d post-harvest segments of growing season in the US (Fig. S7 ). Season-long weather conditions have been used in previous studies 13 , 24 , and it has been shown that choice of growing season does not affect climate-related effects on crop yield 25 , 26 .
As an additional sensitivity analysis, we developed ALE plots for the algorithms using the aforementioned 30-d weather variables (Fig. S8 ). For major management practices, there were no differences in simulated responses between the algorithms that used multiple 30-d weather variables and the final chosen algorithms that used longer intervals (Fig. 3 ). Repeating the analysis for the same hypothetical cropping system in the same Wisconsin location using the algorithms developed with the 30-d weather conditions, the observed trends were consistent with the season-long weather algorithms, although the simulated yields were numerical different (Fig. S9 ). Nevertheless, across all representations of weather conditions (algorithms with 30-d intervals and season-long), the levels/rates of management practices in the 5% highest and lowest yielding maize and 5% highest soybean cropping systems with early sowing date were identical, apart from manure use in maize. Based on these results, we consider the algorithm-derived yield estimates robust to different representations of seasonal weather variability.
It appears that several different cropping systems can result in similar high yield for both crops (Fig. 4 C,D,G,H). This is in agreement with other agricultural decision maker tools 27 . Moreover, it is common for neighboring farms to attain similar crop yield despite the use of a different cropping system, suggesting that a single optimal solution does not necessarily exist and that different combinations of management practices, when they interact with environment, can still result in similar high yields. Since the effect of environment is ever-changing, the high level of complexity of synergies between G × E × M suggests that long-term optimization of single management factor may not be possible 28 , which further highlights the importance of accounting for the effect of the entire cropping system at the field level.
The approach we present here should not be considered as a crop yield forecasting exercise. There have been several attempts to forecast crop yields using deep neural network methods (e.g., 29 , 30 ). In contrast, the algorithms we present here can generate hypothetical experimental data that can be used to rapidly examine G × E × M interaction for both maize and soybean across the US. Of the millions of possible G × E × M combinations, our ML algorithms can identify hidden complex patterns between G × E × M combinations for yield optimization that may be non-obvious, but once identified, worthy of field test confirmation. Farmers can use the algorithms to gain insights about optimum management interactions in their location-specific environment (known soil type × expected weather conditions), and to identify farm factors that may be too costly to alter without a priori reason (generated by the model) for doing so. Researchers can compare expected yield across thousands of hypothetical cropping systems and use the results as a guide to design more efficient future field-based crop management practice evaluation experiments.
We note that this approach should not be considered as a substitute of replicated trials. To the contrary, replicated field trials performed by Universities are continually needed to serve as an excellent source of high-quality unbiased data which can be used to train even more comprehensive algorithms. The major issue with current performance trial data is that a great amount of management information is not reported. Usually, only information relevant to the examined management factors in each trial are reported, which inevitably results in missing values (Tables S1 and S2 ), or even in absence of important variables (e.g., number and dates of split fertilizer application). As we have highlighted here, the high order and complex background management interactions should not be considered as irrelevant.
Conclusions
Agricultural experiments repeated every year in hundreds of locations across the US generate a vast amount of crop yield and management datasets which are useful for broad inferences (average effect of a management practice across a range of environments). Such datasets have, to date, remained disconnected from each other, and are difficult to combine, standardize, and properly analyze. In the presented work, we overcame these issues by developing large databases and by leveraging the power of ML algorithms. We argue that our algorithms can advance agricultural research and aid in revealing a currently hidden yield potential in each individual farm across the US.
Crop yield and management data were obtained from publicly available variety performance trials which are typically performed yearly in several locations across each state 31 . Recorded, trial-specific, management practices for maize included use of irrigation, tillage practice, seeding rate, row spacing, sowing date, previous crop, fertilizer (N, P, and K), use of manure, cultivar’s maturity, insecticide traits and use of seed treatments (Table S1 ). For soybean, use of irrigation, foliar fungicide, tillage practice, seeding rate, row spacing, sowing date, previous crop, and cultivar maturity were recorded (Table S2 ).
Since data were collected from different states and years, it was assumed that reported management practices (general categories) were consistent across all locations. Additionally, the type and application method of fertilizer was rarely reported. Similarly, there was a lack of information on the active ingredient and rates of seed treatments and foliar applied products. We acknowledge that this lack of information, as we state in the discussion section, is a limitation of our databases and our assumption, that the way different management practices are reported across different states is consistent, may have contributed to the observed unexplained variability.
For both databases, data entry was performed manually. Additionally, for both crops, soil type was recorded and weather data (Table S7 ) were retrieved from the DAYMET 32 database for each year and set of coordinates. DAYMET daily data are reasonably accurate when means or totals are calculated over extended periods 33 . Therefore, means and sums for three periods (90–150, 151–270, and 271–330 days of year) (Tables S1 and S2 ) and 30-d periods (Tables S8 and S9 ) were calculated. The different sets of weather variables were used in different models to assess their impact in model accuracy.
The exact coordinates for each site were not reported in the trial reports. Therefore, approximate coordinates, based on the nearest reported city, were used for each unreported site. When unmanageable production adversities were reported (e.g., hail, damage due to deer etc.), the associated data were not used. Missing values were present in almost all management-related variables in both databases (Tables S1 and S2 ). Since the data were derived from designed experiments, levels of management were not a result of response to external factors (e.g., weather conditions) but were researcher’s decisions to answer specific research questions (e.g., crop yield response to different sowing dates or maturity ratings), no missing data imputation was performed.
The first step before data analysis was to examine correlations among the weather variables. Due to their strong collinearity (Figs. S3 and S4 for maize and soybean, respectively), only those with Pearson r < 0.7 were retained for subsequent analyses. The final maize database included seven weather variables (Table S1 ) and the final soybean database included eight weather variables (Table S2 ). Categorical variables were one-hot encoded and then databases were separated in training (80% of database) and testing (20% of database) datasets. To ensure adequate representation of growing environments in both, the training and testing portions of the data, stratified sampling was performed by year, use of irrigation, and soil type. For each crop, an extreme gradient boosting (XGBoost) algorithm 34 was trained to predict final yield as a response of the aforementioned weather and management variables listed in Tables S1 and S2 . The hyperparameters were optimized using the training dataset and included number of estimators, tree depth, number of leaves, minimum sum of instance weight in node, learning rate, subsample percentage, column sample by tree and by level, gamma, alpha and lambda parameters. To efficiently tune the hypermeters, Bayesian optimization was performed using “hyperopt” in Python 3.6.9 with tenfold cross validation. The combination of the hypermeters that resulted in the lowest root mean square error (RMSE) in the tenfold cross validations was chosen as the final model which was further evaluated on the test portion of the data (Fig. 2 in main document).
Accumulated local effects (ALE) plots 14 , which are robust to correlation among independent variables, were developed for indicative and important variables using 1000 Monte Carlo simulations. These plots are useful to visualize how individual features influence the predictions of the developed “black-box” algorithms. To perform the evaluation for the “what if” scenarios, the final algorithms were applied on hypothetical cropping systems in a randomly chosen field in south central Wisconsin (latitude = 43.34, longitude = − 89.38) and weather conditions in 2016–2018 for maize and 2014–2018 for soybean. Boxplots were used to visually evaluate the results.
Data and code availability
The datasets generated during and/or analyzed during the current study are available from the corresponding author on reasonable request.
Godfray, H. C. J. et al. Food security: The challenge of feeding 9 billion people. Science 327 , 812–818 (2010).
Article ADS CAS Google Scholar
Schlenker, W. & Lobell, D. B. Robust negative impacts of climate change on African agriculture. Environ. Res. Lett. 5 , 014010 (2010).
Article ADS Google Scholar
Mourtzinis, S. et al. Climate-induced reduction in US-wide soybean yields underpinned by region- and in-season specific responses. Nat. Plants 1 , 14026 (2015).
Article Google Scholar
Hoffman, L. A., Kemanian, A. R. & Forest, C. E. The response of maize, sorghum, and soybean yield to growing-phase climate revealed with machine learning. Environ. Res. Lett. 15 , 094013 (2020).
Folberth, C. et al. Uncertainty in soil data can outweigh climate impact signals in global crop yield simulations. Nat. Commun. https://doi.org/10.1038/ncomms11872 (2016).
Article PubMed PubMed Central Google Scholar
Makinen, H., Kaseva, J., Virkajarvi, P. & Kahiluoto, H. Shifts in soil–climate combination deserve attention. Agric. For. Meteorol. 234 , 236–246 (2017).
Rattalino Edreira, J. I. et al. Assessing causes of yield gaps in agricultural areas with diversity in climate and soils. Agric For Meteorol 247 , 170–180 (2017).
Mourtzinis, S. et al. Sifting and winnowing: analysis of farmer field data for soybean in the US North-Central region. Field Crops Res. 221 , 130–141 (2018).
Pradhan, P., Lüdeke, M. K. B., Reusser, D. E. & Kropp, J. P. Food self-sufficiency across scales: How local can we go?. Environ. Sci. Technol. 48 , 9463–9470 (2014).
Frieler, K. et al. Understanding the weather signal in national crop-yield variability. Earths Fut. 5 , 605–616 (2017).
Puntel, L. A. et al. Modeling long-term corn yield response to nitrogen rate and crop rotation. Front. Plant Sci. 7 , 1630 (2016).
Rong, J. et al. Exploring management strategies to improve maize yield and nitrogen use efficiency in northeast China using the DNDC and DSSAT models. Comput. Electron. Agric. 166 , 104988 (2019).
Leng, G. & Hall, J. W. Predicting spatial and temporal variability in crop yields: an inter-comparison of machine learning, regression and process-based models. Environ. Res. Lett. 15 , 044027 (2020).
Apley, D. W. & Zhu, J. Visualizing the effects of predictor variables in black box supervised learning models. arXiv:1612.08468v2 (2016).
Swanson, S. P. & Wilhelm, W. W. Planting date and residue rate effects on growth, partitioning, and yield of corn. Agron. J. 88 , 205–210 (1996).
Wiatrak, P. J. & Wright, D. Corn hybrids for late planting in the Southeast. Agron. J. 96 , 1118–1124 (2004).
Bruns, H. A. & Abbas, H. K. Planting date effects on Bt and non-Bt corn in the mid-south USA. Agron. J. 98 , 100–106 (2006).
Long, N. V., Assefa, Y., Schwalbert, R. & Ciampitti, I. A. Maize yield and planting date relationship: A synthesis-analysis for US high-yielding contest-winner and field research data. Front. Plant Sci. 8 , 2106 (2017).
Mourtzinis, S., Specht, J. E. & Conley, S. P. Defining optimal soybean sowing dates across the US. Sci. Rep. 9 , 2800 (2019).
Assefa, Y. et al. Yield responses to planting density for US modern corn hybrids: A synthesis-analysis. Crop Sci. 56 , 2802–2817 (2016).
Article CAS Google Scholar
Light, M. A., Lenssen, A. W. & Elmore, R. W. Corn (Zea mays L.) seeding rate optimization in Iowa, USA. Precis. Agric. 18 , 452–469 (2016).
Gaspar, A. et al . Defining optimal soybean seeding rates and associated risk across North America. Agron. J. 1–12 (2020).
Andrade, J. et al. Assessing the influence of row spacing on soybean yield using experimental and producer survey data. Field Crops Res. 230 , 98–106 (2019).
Lobell, D. B. et al. The critical role of extreme heat for maize production in the United States. Nat. Clim. Change 3 , 497–501 (2013).
Lobell, D. B. & Field, C. B. Global scale climate–crop yield relationships and the impacts of recent warming. Environ. Res. Lett. 2 , 014002 (2007).
Schlenker, W. & Roberts, M. J. Nonlinear temperature effects indicate severe damages to US crop yields under climate change. Proc. Natl. Acad. Sci. 106 , 15594–15598 (2009).
Hochman, Z. et al. Re-inventing model-based decision support with Australian dryland farmers. 4. Yield prophet (R) helps farmers monitor and manage crops in a variable climate. Crop Pasture Sci. 60 , 1057–1070 (2009).
Sadras, V. O. & Densison, R. F. Neither crop genetics nor crop management can be optimized. Field Crops Res. 189 , 75–83 (2016).
Khaki, S. & Wang, L. Crop yield prediction using deep neural networks. Front. Plant Sci. 10 , 621 (2019).
Khaki, S., Wang, L. & Archontoulis, S. V. A CNN-RNN framework for crop yield prediction. Front. Plant Sci. 10 , 1750 (2020).
Websites for each state-specific university variety trial can be found in Table S10 in supplementary material.
Thornton, P.E. et al. Daymet: Daily surface weather data on a 1-km grid for North America, Version 3. ORNL DAAC, Oak Ridge, Tennessee, USA. https://doi.org/10.3334/ORNLDAAC/1328 (2016).
Mourtzinis, S., Rattalino Edreira, J. I., Conley, S. P. & Grassini, P. From grid to field: assessing quality of gridded weather data for agricultural applications. Eur. J. Agron. 82 , 163–172 (2017).
Chen, T. & Guestrin, C. XGBoost: A Scalable tree boosting system. arXiv:1603.02754v3 (2016).
Download references
Acknowledgements
The authors thank Adam Roth and multiple students for their help in database development and John Gaska for constructing Fig. 1 . This research was funded in part by the Wisconsin Soybean Marketing Board, The North Central Soybean Research Program (S.P. Conley), and the USDA National Institute of Food and Federal Appropriations under Project PEN04660 and Accession number 1016474 (P.D. Esker).
Author information
Authors and affiliations.
Agstat Consulting, Athens, Greece
Spyridon Mourtzinis
Department of Plant Pathology and Environmental Microbiology, Pennsylvania State University, State College, PA, 16801, USA
Paul D. Esker
Department of Agronomy and Horticulture, University of Nebraska-Lincoln, Lincoln, NE, 68583-0915, USA
James E. Specht
Department of Agronomy, University of Wisconsin-Madison, Madison, WI, 53706, USA
Shawn P. Conley
You can also search for this author in PubMed Google Scholar
Contributions
S.M. conceived the idea, analyzed the data, and wrote the paper. P.D.E and J.E.S. contributed to idea development, reviewed results, and provided revisions for improvement of the manuscript. S.P.C. contributed to the data set and idea development, reviewed results, and commented on the manuscript.
Corresponding author
Correspondence to Spyridon Mourtzinis .
Ethics declarations
Competing interests.
The authors declare no competing interests.
Additional information
Publisher's note.
Springer Nature remains neutral with regard to jurisdictional claims in published maps and institutional affiliations.
Supplementary Information
Supplementary information., rights and permissions.
Open Access This article is licensed under a Creative Commons Attribution 4.0 International License, which permits use, sharing, adaptation, distribution and reproduction in any medium or format, as long as you give appropriate credit to the original author(s) and the source, provide a link to the Creative Commons licence, and indicate if changes were made. The images or other third party material in this article are included in the article's Creative Commons licence, unless indicated otherwise in a credit line to the material. If material is not included in the article's Creative Commons licence and your intended use is not permitted by statutory regulation or exceeds the permitted use, you will need to obtain permission directly from the copyright holder. To view a copy of this licence, visit http://creativecommons.org/licenses/by/4.0/ .
Reprints and permissions
About this article
Cite this article.
Mourtzinis, S., Esker, P.D., Specht, J.E. et al. Advancing agricultural research using machine learning algorithms. Sci Rep 11 , 17879 (2021). https://doi.org/10.1038/s41598-021-97380-7
Download citation
Received : 17 January 2021
Accepted : 25 August 2021
Published : 09 September 2021
DOI : https://doi.org/10.1038/s41598-021-97380-7
Share this article
Anyone you share the following link with will be able to read this content:
Sorry, a shareable link is not currently available for this article.
Provided by the Springer Nature SharedIt content-sharing initiative
This article is cited by
Various tomato infection discrimination using spectroscopy.
- Bogdan Ruszczak
- Krzysztof Smykała
- Pedro Javier Navarro Lorente
Signal, Image and Video Processing (2024)
Machine learning in agriculture: a review of crop management applications
- Ishana Attri
- Lalit Kumar Awasthi
- Teek Parval Sharma
Multimedia Tools and Applications (2023)
A robust and resilience machine learning for forecasting agri-food production
- Amin Gholamrezaei
- Kiana Kheiri
Scientific Reports (2022)
By submitting a comment you agree to abide by our Terms and Community Guidelines . If you find something abusive or that does not comply with our terms or guidelines please flag it as inappropriate.
Quick links
- Explore articles by subject
- Guide to authors
- Editorial policies
Sign up for the Nature Briefing newsletter — what matters in science, free to your inbox daily.

The Mission of Agricultural Research
Cite this chapter.
- Gad Loebenstein 3 &
- George Thottappilly 4
815 Accesses
3 Citations
Agricultural research seems to be the oldest form of organized research in the world. Agricultural research can be broadly defined as any research activity aimed at improving productivity and quality of crops by their genetic improvement, better plant protection, irrigation, storage methods, farm mechanization, efficient marketing, and a better management of resources.
This is a preview of subscription content, log in via an institution to check access.
Access this chapter
Subscribe and save.
- Get 10 units per month
- Download Article/Chapter or eBook
- 1 Unit = 1 Article or 1 Chapter
- Cancel anytime
- Available as PDF
- Read on any device
- Instant download
- Own it forever
- Compact, lightweight edition
- Dispatched in 3 to 5 business days
- Free shipping worldwide - see info
- Durable hardcover edition
Tax calculation will be finalised at checkout
Purchases are for personal use only
Institutional subscriptions
Unable to display preview. Download preview PDF.
Similar content being viewed by others
Agricultural Research
A Decade of Agricultural Research in Rwanda: Achievements and the Way Forward
Public Research and Technology Transfer in US Agriculture: The Role of USDA
Aldrich, D.G. 1966. Agricultural Research, a Key to Understanding our Environment. In: Symposium on Research in Agriculture . USDA, Agricultural Research Service, Washington, DC, pp. 50-53.
Google Scholar
Asopa, V.N., and Beye, G. 1997. Management of Agriculture Research. A Training Manual. FAO. Rome.
Anonymous 1999. INRA, from the molecule to the marketplace. INRA Scientific Information and Communication Directorate.
Asopa, V.N., and Beye, G. 1977a. Institutional Agricultural Research: Organization and Management. Module 1 Exhibit 3. FAO, Rome, Italy.
Asopa, V.N., and Beye, G. 1977b. Institutional Agricultural Research: Organization and Management. Module 2 . FAO, Rome, Italy.
Download references
Author information
Authors and affiliations.
Agricultural Research Organization, Volcani Center, 50-250, Bet Dagan, Israel
Professor Gad Loebenstein
Biotechnology, Sahrdaya College of Engineering and Technology, 680 684, Thrissur, Kerala, India
Dean George Thottappilly
You can also search for this author in PubMed Google Scholar
Editor information
Editors and affiliations.
Volcani Center, 50-250, Bet Dagan, Israel
Gad Loebenstein
Sahrdaya College of Engineering and Technology, 680 684, Thrissur, Kerala, India
George Thottappilly
Rights and permissions
Reprints and permissions

Copyright information
© 2007 Springer
About this chapter
Loebenstein, G., Thottappilly, G. (2007). The Mission of Agricultural Research. In: Loebenstein, G., Thottappilly, G. (eds) Agricultural Research Management. Springer, Dordrecht. https://doi.org/10.1007/978-1-4020-6057-1_1
Download citation
DOI : https://doi.org/10.1007/978-1-4020-6057-1_1
Publisher Name : Springer, Dordrecht
Print ISBN : 978-1-4020-6056-4
Online ISBN : 978-1-4020-6057-1
eBook Packages : Biomedical and Life Sciences Biomedical and Life Sciences (R0)
Share this chapter
Anyone you share the following link with will be able to read this content:
Sorry, a shareable link is not currently available for this article.
Provided by the Springer Nature SharedIt content-sharing initiative
- Publish with us
Policies and ethics
- Find a journal
- Track your research
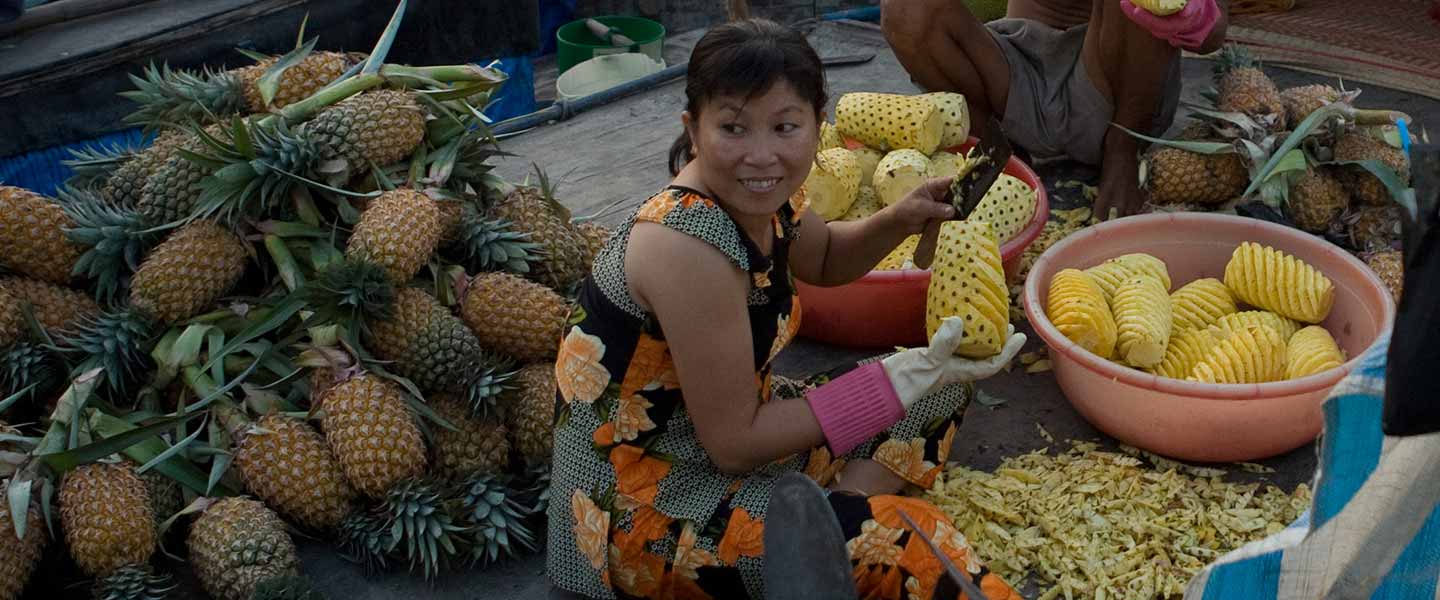
Agriculture and Food
Agriculture can help reduce poverty, raise incomes and improve food security for 80% of the world's poor, who live in rural areas and work mainly in farming. The World Bank Group is a leading financier of agriculture.
Healthy, sustainable and inclusive food systems are critical to achieve the world’s development goals. Agricultural development is one of the most powerful tools to end extreme poverty, boost shared prosperity, and feed a projected 10 billion people by 2050 . Growth in the agriculture sector is two to four times more effective in raising incomes among the poorest compared to other sectors.
Agriculture is also crucial to economic growth: accounting for 4% of global gross domestic product (GDP) and in some least developing countries, it can account for more than 25% of GDP .
But agriculture-driven growth, poverty reduction, and food security are at risk: Multiple shocks – from COVID-19 related disruptions to extreme weather, pests, and conflicts – are impacting food systems. The goal of ending global hunger by 2030 is currently off track. Conflicts, climate change, and high food prices are driving food and nutrition insecurity, pushing millions into extreme poverty, and reversing hard-won development gains. Around a quarter of a billion people now face acute food insecurity .
The growing impact of climate change could further cut crop yields, especially in the world’s most food-insecure regions. At the same time, our food systems are responsible for about 30% of greenhouse gas emissions.
Current food systems also threaten the health of people and the planet and generate unsustainable levels of pollution and waste. One third of food produced globally is either lost or wasted. Addressing food loss and waste is critical to improving food and nutrition security, as well as helping to meet climate goals and reduce stress on the environment.
Risks associated with poor diets are also the leading cause of death worldwide. Millions of people are either not eating enough or eating the wrong types of food, resulting in a double burden of malnutrition that can lead to illnesses and health crises. Food insecurity can worsen diet quality and increase the risk of various forms of malnutrition, potentially leading to undernutrition as well as people being overweight and obese. An estimated 3 billion people in the world cannot afford a healthy diet.
Last Updated: Mar 15, 2024
The World Bank Group provides knowledge, advice, and financial resources in low- and middle-income countries to transform food systems to reduce poverty and achieve green, resilient, and inclusive development.
Our work in food and agriculture focuses on:
- Food and nutrition security , where we work with efforts to share information, and to rapidly provide resources where they are needed, while helping countries design the long-term reforms needed to build resilient food and nutrition systems.
- Climate-smart agriculture by working with client governments to provide solutions that address global climate priorities, while recognizing national contexts and development objectives.
- Data-driven digital agriculture by expand the frontier of financing and expertise for digital agriculture.
- Mobilizing capital for development in agriculture & food . We identify and leverage growth areas for productive investments, focusing on innovation and impact. And we design projects to ensure that financing boosts sustainable productivity gains, reaches smallholders and SMEs, and creates jobs to end poverty and hunger.
- Public policy and expenditure by working with governments to facilitate the adoption of more sustainable approaches, technologies, and practices, alongside policies that promote public and private sector investment.
- Sustainable and healthy diets to ensure that food can support a healthy population.
In fiscal year 2024, the World Bank committed US$3 billion in new financing in 2024 – with over 41% of all commitments for Sub-Saharan Africa.
Increasingly, the Bank supports country efforts to transform their food systems by taking a holistic look at public policies and spending for agriculture and food. A Multi-Donor Trust Fund, Food Systems 2030 , provides a platform for change in this area.
Last Updated: Aug 20, 2024
In Angola, a project co-financed by the World Bank and the French Agency for Development, contributed to the government economic diversification agenda by supporting the transition from subsistence to a more market-oriented, competitive agriculture sector. The project is helping producers or small and medium enterprises prepare and finance agriculture investments. As of December 2023, 268 projects have been approved, equivalent to about $37 million in agriculture investment. The project funded the first partial credit guarantees scheme ever dedicated to the agriculture sector in Angola – an innovation for the country’s agribusiness sector – mobilizing so far $4.1 million in private bank financing.
In Argentina , the Bank supported 14,630 families who benefited from better socioeconomic inclusion. Under the project, 2,409 families accessed water for human and animal consumption, also irrigation; 7,499 rural families improved their productive capacity; and over 900 families accessed infrastructure, equipment and training that improved their marketing. Based on the model of productive alliances, 2,801 families from different regions became beneficiaries by linking their production with the markets. Among the funded activities, the production of honey, orchards, forage, livestock, nuts, spices, yerba mate and tea, among others, stand out.
In Benin, between 2011-2021, the Agricultural Productivity and Diversification Project facilitated the adoption of productivity-enhancing technologies for 327,503 crop producers, leading to 135,549 hectares of land cultivated with improved technologies. The project interventions resulted in increased yields from 0.45 ton to 0.81 ton for cashew; from 1.2 tons to 2.97 tons for maize, from 4 tons to 6.2 tons for rice, and from 50 tons to 70 tons for pineapple. The project led to significant increases of milled rice and fish output. Combined with support for crop production and processing, support to exports has led to increases in the export of cashew and pineapple.
For the past 18 years, Bolivia has been developing a strategy to improve agricultural production and marketing through the productive alliances model. This model links small rural producers with markets, and facilitates their participation in value chains, and access to technical assistance and technology for better market access. Currently, over 2,600 productive alliances have been implemented, benefiting 107,308 producer families. In 2023, the third phase of productive alliances model was launched, expecting to have a significant impact on nearly 130, 000 rural producer’s communities, with a focus on food security, adoption of innovative practices for resilient agriculture and the increased participation of women producers.
A Bank-supported project implemented in partnership with the Government of Rio Grande do Norte, one of Brazil's poorest and most violent states, has aimed to improve agricultural productivity, the quality of and access to health, public security, education and public sector management across the state. The project has implemented 131 subprojects in family farming, renovated 274km of roads, renovated and strengthened the safety of an important dam, and built 22 modern, multi-service Citizen Centers.
In Bhutan, a project is supporting the government's efforts to reduce rural poverty and malnutrition through climate-smart agriculture. Irrigation technology and greenhouses introduced through the project have helped farmers to increase their access to local and export markets. More than 6,500 people have increased the quality and quantity of produce like rice, maize, potato, vegetables, quinoa, citrus, apples, and potatoes, as well as high-value spices such as cardamom and ginger.
In Burkina Faso, the Bank supported the Burkina Faso Livestock Sector Development Project which ran from 2017 to 2022. By project completion, beneficiaries among selected value chains increased their yield by 8.4%. Yield increase for cattle, sheep, and egg production reached 6.76%, 11.93%, and 6.50%, respectively. Sales increased by 45% exceeding the target of a 30% increase. The volume of loans granted by partner financial institutions reached $5.02 million, exceeding the original target of $4.38 million. The project reached a total of 329,000 beneficiaries, out of which 138,314 were women and 112,573 were youth.
In the Central African Republic, through the Emergency Food Security Response project, 330,000 smallholder farmers received seeds, farming tools, and training in agricultural and post-harvest techniques. The project helped farmers boost their crop production and become more resilient to climate and conflict risks. Local food production increased by 250%, from 28,000 tons in September 2022 to 73,000 tons in June 2023. Moreover, 21,006 agricultural households received training on post-harvest loss management and provided equipment, such as mobile storage units, to enhance packaging of agricultural products, leading to higher selling prices.
In Colombia, since 2010, the adoption of environmentally friendly silvopastoral production systems (SPS) for over 4,100 cattle ranches has converted 100,522 hectares of degraded pastures into more productive landscapes and captured 1,565,026 tons of CO2 equivalent. In addition, almost 40,000 hectares of pastureland were transformed to SPS and 4,640 hectares into intensive Silvopastoral Production Systems (iSPS). Moreover, 4,100 direct farmers beneficiaries, of which 17% were women, were trained in SPS and iSPS, and over 21,000 farmers, technicians and producers were also trained, visited demonstration farms, and participated in workshops and events and technology brigades. A network of 116 plant nurseries were also established, which produced around 3.1 million fodder trees that were delivered to beneficiary farmers.
In Cote d’Ivoire, between 2013 and 2017, the Agriculture Sector Project boosted the productivity of 200,000 farmers and rehabilitated 6,500 kilometers of rural roads allowing farmers to better transport their products and reduce post-harvest losses. To aid the cashew industry, the Bank also supported a research program that helped disseminate 209 genotypes of high-performing trees and establish 18 nurseries. The Bank-financed project also helped leverage $27.5 million in private investment to boost productivity on at least 26,500 hectares.
In Ethiopia, since 2015 a project has helped 2.5 million smallholder farmers increase agricultural productivity and commercialization by establishing market linkages, increasing access to agricultural public services, building smallholder farmer capacity in efficient water and crop management to implement climate change mitigation and adaptation, and improving diet diversification. The project has also been promoting the use of nutrition sensitive agriculture and gender and climate-smart agriculture including dietary diversity through nutrient-dense crops, livestock products, post-harvest processing/handling and social behavioral change communication, along with food safety and child and maternal health. The project has supported farmers increase yield in crops and livestock by 19% and 52% respectively and their revenue by 96.2%. To date the project has also provided 58,823 hectares of land with irrigation and water related services, and over 1.6 million farmers have adopted improved agriculture technologies promoted by the project. Nearly one million jobs for rural people, including for women and youth in fragile and conflict affected areas have been created as a result of the project interventions.
In Grenada , the World Bank supported local farmers and fisherfolk, along with aggregators and agro-processors to enhance their access to markets and sales from 2017 to 2023 through the OECS Regional Agriculture Competitiveness Project. The project provided vouchers to 206 farmers and fisherfolk and offered co-financing opportunities for 10 agro-processors, leading to significant improvements in their production facilities and market access. Additionally, 260 employees and 53 extension workers received training, improving their skills in agricultural production and market reach. Through the project, 150 producers adopted various climate-smart technologies, such as solar panels and rainwater harvesting systems, underscoring the project's dedication to sustainability and efficiency.
In Guinea, from 2018 to 2023, through the Guinea Integrated Agricultural Development Project , local farmers increased agriculture's productivity, and sustainability. To help local communities, the project disseminated high-yielding seeds, improve irrigation, and trained women and youth to access funds to create jobs. The project also promoted the use of climate-smart, gender-sensitive digital technologies with local producers. The project has reached 149,000 farmers (of whom 38% are women and 30% are youth). The project’s results include a 30% increase in yield of rice and maize; a 42% increase in commodity sales; a 47,470-hectare area covered by improved technologies; over 97,000 users of improved technologies, and more than 2,000 jobs created for women and youth.
In Haiti , a World Bank project strengthened the institutional capacity of Haiti’s Ministry of Agriculture and Rural Development by accessing technologies to increase not only agricultural productivity and production but also improved livelihoods and resilience. The project developed irrigation and drainage on 2,244 hectares; established 115 farmer field schools, and trained facilitators in agricultural extension techniques. A total of 78,242 small producers increased their market access, half of whom were women; more than 3,368 private and public sector staff (including staff from the Ministry of Agriculture, municipal staff, among others) and 600 farmers were trained on surveillance and vaccination, the use of fruit fly traps, mealybugs control, and protection of animals against rabies and anthrax and more than 3.6 million animals were vaccinated.
In Honduras, since 2010 , 12,878 small farmers, of which 27% are women, have used productive alliances to improve productivity and access to markets, which has leveraged $33.5 million in finance from commercial banks and microfinance institutions. Under the project, gross sales of producer organizations rose by 25.3%. Also, support to Honduras’ Dry Corridor Alliance, has helped 12,202 households implement food security and agricultural business plans, and improved agricultural yields, nutrition, and food diversity of project beneficiaries.
In India, the Assam Agribusiness and Rural Transformation Project supported over 400,000 farm families and 1,270 businesses and over 100 of industry associations and producer organizations in improving their productivity and incomes and helping develop new marketing channels since 2017.
In Kenya, since 2016, 1.5 million farmers , where over 60% are women, have increased their productivity , climate resilience and access to markets. The digital registry (including geo tagging) of these 1.5 million farmers enables them to access agro-weather and market advisories. In addition, the Bank is facilitating partnerships between the government and 26 ag-tech support agencies which enables almost 500,000 farmers to access a range of services (inputs, financial services and markets) by leveraging digital technologies.
In Kosovo , the Bank provided 775 grants to farmers and 103 grants to agri-processors to increase production capacities and enhance market competitiveness in the livestock and horticulture sector. This was done through upgrading facilities, adopting new technologies, and introducing food safety and environmental standards. Further, support was provided for the rehabilitation of irrigation schemes covering an area of 7,750 hectares which had an impact on the production, yield, quality, and variety of products cultivated in the area.
In the Kyrgyz Republic, the Additional Financing to the Integrated Dairy Productivity Improvement Project is improving productivity through better technologies and breeds of dairy animals rather than increasing their numbers. The project provides training, artificial insemination services, and monitoring milk yields per cow and the quality of milk to processing companies. To date, 10,000 small farmers including 5,000 women farmers, have received training to enhance productivity and climate-smart agriculture. Over 13,000 cows received artificial insemination for breed improvement with positive pregnancy rate of 67.3% which is above the global average. With improved breeds of dairy animals, the market value of the crossbred calves is higher than local calves and the average milk yield per cow has increased by nearly 15%. The project has also established a digital tool to monitor milk quality which is being used by eight dairy processing companies. The project established 30 milk collection points through famers’ Jamaats that are equipped with refrigerated tanks and advanced testing equipment, strategically located to ensure consistent milk quality and timely delivery, especially during hot summers.
In Madagascar, since 2016 , the Bank has boosted the productivity of over 130,000 farmers. Sixty-thousand hectares of irrigated rice fields have been rehabilitated. The Bank also supported the cocoa sector through research, the development of certified seeds, and promotion of improved production and processing techniques. This allowed 4,000 cocoa producers to increase their incomes and increase production and export volumes by 50%. The Bank also financed the country’s largest land rights registration, facilitating the delivery of over 200,000 land certificates to farmers.
In Mauritania, between 2016 and 2021, the intervention of the Sahel regional support project offered agricultural assets and services to more than 400,000 farmers/pastoralists, where nearly 30% are women. More than 1.9 million hectares of land under sustainable management practices, in addition to the construction of 133 vaccination parks and the realization of 118 water points (wells and boreholes) as well as other infrastructure of valorization and trade of animals were provided to agro-pastoralist communities. Additionally, from April 2023- June 2028, the Bank offered to support the Agriculture Development and Innovation Support Project (PADISAM) to improve land resources management and foster inclusive and sustainable commercial agriculture in selected areas of Mauritania. It is anticipated that by the end of the project, there will be 72,000 direct beneficiaries and about 5,000 Ha of land under sustainable landscape management practices.
Following Russia’s invasion of Ukraine and the resulting spikes in wheat prices in 2022, the World Bank provided emergency support to several countries in the Middle East and North Africa to mitigate the negative socio-economic consequences on the poor and vulnerable. These emergency projects secured access to affordable bread for over 89 million people across the region. In Lebanon, a project ($150 million) has been financing wheat imports that supports universal access to affordable Arabic bread for over a year to 5.36 million people living in Lebanon, of which 1 million are Syrian, Palestinian, and other refugees. In Egypt, a project helped procure around 1.15 million metric tons of wheat – equivalent to at least a 2-month supply to cover the needs of 72 million vulnerable people. A project in In Tunisia procured 160,099 metric tons of soft wheat, equivalent to seven weeks of bread supply for a population of 12 million.
In Moldova, since 2012 , the Bank has helped more than 7,500 farmers gain access to local and regional high-value markets for fresh fruit and vegetables and boosted land productivity through the promotion of sustainable land management practices on 120,000 hectares of farmland.
In Montenegro , the bank, through the Second Institutional Development and Agriculture Strengthening (MIDAS2), helped the government launch the very first Instrument for Pre-accession Assistance for Agriculture and Rural Development (IPARD)-like agro-environmental measure in a manner compliant with EU requirements, increasing the amount of meadows and pasture lands recorded in the Land Parcel Identification System (LPIS) from 13,600 hectares (ha) to 92,000 ha. The Bank has also supported almost 4,000 farmers working on orchards, vineyards, livestock and aromatic plants, 224 agro-processors, and 59 farmers working on processing on-farm complying with the European Union requirements for food safety and 278 agricultural households adopting agro-environmental measures, improving their competitiveness and sustainability.
In Morocco , the Strengthening Agri-food Value Chains Program for Results has financed the construction of the first modern regional wholesale market in Rabat, which will improve the distribution of agricultural products throughout the region, benefiting more than 4.6 million inhabitants. The program also financed the establishment of the male sterile Ceratite production center, which will enable citrus producers in the Souss-Massa and Berkane regions, which represent 52% of national citrus production and generate about 6 million working days per year, to protect their production from damage caused by the Mediterranean fruit fly. The program also enabled more than 1,000 agri-food SMEs to obtain sanitary approval after upgrading, leading to an increase in employment by almost 61%. The program co-financed more than 70 units of packaging, cold storage and processing, which leveraged about US$86 million as private investment and led to an overall increase in production value of around 34%.
In Niger , through the Climate Smart Agriculture Support Project , the World Bank supported over 370,000 farmers, where 145,000 of whom are women. The farmers benefited from the project’s investments in small and large-scale irrigation, improved climate-smart agriculture, and sustainable land management practices. Over 154,000 hectares of land were developed with sustainable land management practices, and 4,400 hectares of cropland were brought under irrigation. In collaboration with the International Crops Research Institute for the Semi-Arid Tropics and FAO, the project promoted good agriculture practices through farmer led e-extension services and technical assistance. The project investments led to significant increases in agriculture productivity: yields of cowpea, millet, and sorghum increased by 169, 164, and 142 percent, respectively. The project also strengthened the national climate information system by building the capacity of the National Meteorology Department (the project installed 30 meteorological stations and 600 rain gauges). Through its support to the Sahel Regional Center for Hydro and Agrometeorology, the project strengthened the early warning systems of national institutes such as National Meteorology and the National Hydrology Directorate.
In Nigeria, APPEALS Project was designed to enhance agricultural productivity of small and medium scale farmers and improve value addition along priority value chains. Since 2017, the project has demonstrated 204 improved technologies to 93,000 farmers. Food crop production has surged, with 304,516 metric tons produced, representing 3.1% of the national output. Furthermore, the project has reached 61,171 farmers with processing assets to improve the quality of their produce. The project also trained 10,346 women and youth, including persons with disability, providing them with business, technical and life skills training, support to business planning and facilitation of business name registration, start-up grant to establish a commercially viable business, and mentorship to provide the beneficiaries with continued support from established agribusiness entrepreneurs. The project linked farmers to market through the facilitation of commercial partnerships resulting in a total of 327 business alliances with 147 off-takers already buying farmers’ produce across the 11 value chains, with a transaction worth of US$ 59.7 million. Similarly, the project has linked 200 agribusiness clusters to infrastructures which includes 55km rural farm access road, 75 aggregation and cottage processing centers, 102 solar-powered water intervention and energy supplies.
In Paraguay, since 2008, 20,863 farmers increased their agricultural income by at least 30% and 18,951 adopted improved agricultural practices, boosting the productivity of their land.
In the Philippines, since 2015 , the Bank helped raise rural incomes, enhance farm and fishery productivity, improve market access and mainstream institutional and operational reforms, as well as science-based planning for agricultural commodities in 81 provinces. The project has benefitted a total of 323,501 people–46% of them women–with farm roads, irrigation, and agricultural enterprise projects, boosting incomes by up to 36%.
In Rwanda, since 2010, the Bank helped support over 410,000 farmers – half are women – in improving their agricultural production by developing over 7,400 hectares for marshland irrigation, providing hillside irrigation on over 2,500 hectares, and several hundreds of farmers benefitted matching grants to support their investments in Farmer-Led Irrigation Development (FLID) technologies on over 1,200 hectares of their land. Interventions also included improving soil conservation and erosion on more than 39,000 hectares of hillside. Maize, rice, beans, and potato yields have all more than doubled and around 2.5 tons of vegetables are exported to Europe and the Middle-East every week from intervention areas, or locally, where more horticulture produce is sold to premium markets including 5-star hotels or the national airline, RwandAir. Less than two years after one of the Bank supported projects introduced greenhouse farming in its intervention areas to minimize the impacts of unfavorable weather conditions and better manage crop pests and diseases, by 2023, the demand for these technologies has seen a rapid increase in these areas and 132 units have been acquired and installed through the matching grants program under the project. Evidence shows relatively high revenues for farmers investing in greenhouse technology, with revenues increasing up to 15 times for vegetable growers.
Since 2019, the ongoing Serbia Competitive Agriculture Project has been supporting the government economic diversification and competitiveness agenda for small and medium scale farmers and their participation in a more market-oriented agriculture sector. The productive alliance model supported by the project has contributed to the improvement of the agri-food market linkages of 823 farmers, of which 330 are women farmers. Through the project, 4,356 farmers have received technical assistance to prepare their business ideas and plans (1,307 are women), while 1,319 business plans have received support in various forms, such as matching grants, technical assistance, and business development support. The farmers have signed their loans with commercial banks to invest in farm innovations, including equipment, on-farm irrigation, digital agriculture, climate-smart agriculture technologies. By providing co-financing with EUR 24.17 million in matching grants, the project-supported business plans have leveraged an additional EUR 24.17 million in private capital so far, including commercial loans to farmers at market interest rate from 11 local banks, and cash contributions from the beneficiary farmers. Amongst them, 1,117 beneficiary farmers are first-time users of credit.
In Tajikistan , the Bank supported the establishment of 545 farmer groups in horticulture value chains, specifically apricot, apple, pear, lemon, cucumber, and tomato, and dairy value chain benefiting a total of 13,516 farmers out of which 48% were women. The Bank also supported the establishment of 342 productive partnerships benefitting 4,340 smallholder farmers. A total of 21,882 beneficiaries achieved an increase in commercial activity. The project supported training for 13, 516 farmers, on value chain development.
In Tunisia, the Bank helped 113 remote rural villages improve land management practices on 37,000 hectares of land to increase productivity and improve 930 kilometers of rural roads serving some 160 villages.
In Uruguay, since 2014, climate-smart agriculture techniques have been adopted on 2.7 million hectares and adopted by 5,541 farmers, providing for a carbon sequestration potential of up to 9 million tons of CO2 annually.
In Uganda, since 2015 , the Agriculture Cluster Development Project’s e-voucher scheme has leveraged over $12 million of farmer investments enabling over 450,000 farm households access and use improved agro-inputs resulting in higher farm yields. Provision of matching grants has enhanced storage capacity by 55,000MT, acquiring value addition equipment and machinery thereby facilitating Producer Organizations to add value and undertake collective marketing. Additional infrastructure support addressing road chokes has also led to improved market access.
The Bank has also made investments into strengthening regulatory and administrative functions of the Ministry of Agriculture through the development of IT Platforms and tools facilitating timely planning and decision making.
In the Uganda Multi-Sectoral Food and Nutrition Security Project, the Bank has supported enhanced knowledge on nutrition resulting in improved household nutrition and incomes for 1.55 million direct project beneficiaries.
In Uzbekistan, the Horticulture Development Project has helped create, 34,520 jobs, including 13,124 for women; increase beneficiary productivity by 24% and profitability by 124%, including through entry into new export markets. The Livestock Sector Development Project supported a sub-loans benefitting 560 large scale commercial livestock farmers, and a total of 135 value chain development projects benefiting 1,456 smallholder farmers (Dekhans). As a result, the share of improved and high yielding livestock breeds increased by 98.7%; increasing milk and meat productivity by 33% and 38% respectively. The Ferghana Valley Rural Enterprise Project has supported the establishment and operation of nine business incubation hubs in Andijan, Namangan, and Ferghana regions, to support local entrepreneurs in business plan preparation, and facilitated access to finance, technology infusion, also organized training among 5,000 project initiators in 36 districts of Ferghana Valley. The project, under its credit line activities, financed a total of 501 investment sub-projects with $119.6 million of the project fund, of which 77.8% were for small business entrepreneurs This created substantial number of new jobs, and increased the incomes of rural enterprises,
In Vietnam, since 2010, the Bank has promoted sustainable livelihoods by helping develop 9,000 “common interest groups” comprising over 15,500 households and partnering them with agricultural enterprises. The Bank also helped over 20,000 farmers improve their livestock production and benefited an additional 130,000 people through capacity building in food safety.
Under the West African Agricultural Productivity Program , the Bank supported a research and development effort that promoted technology generation, dissemination, and support to local farming systems in 13 ECOWAS countries. The project reached over 2.7 million beneficiaries, 41% of whom were women. It also generated 112 technologies that reached over 1,850,000 hectares.
The Yemen Food Security Response and Resilience Project has directly benefited over 1 million beneficiaries to date. The project is focusing on resilience building amidst protracted crisis – including conflict, insecurity, and climate-related shocks. The project has created around 20,000 short-term jobs and benefited over 50,000 smallholder farm households through various agricultural infrastructure improvements. The project invested in the vaccination of 11 million small ruminants and treated a similar number for parasites. In addition to building resilience, as a short-term response, the project supported 20,000 vulnerable households with kitchen gardens and livestock kits, business development training and start-up grants to vulnerable women. Furthermore, the project facilitated a supplemental feeding program for over 740,000 most vulnerable beneficiaries.
Last Updated: Apr 09, 2024
The World Bank works with a range of partners to achieve ambitious development goals: transforming food systems, boosting food security and empowering smallholder farmers, to realize zero hunger and poverty by 2030.
The World Bank Group is a joint convener, with the G7 Presidency, of the Global Alliance for Food Security (GAFS) . A key outcome of the Global Alliance is the Global Food and Nutrition Security Dashboard , a key tool to fast-track a rapid response to the unfolding global food security crisis, designed to consolidate and present up-to-date data on food crisis severity, track global food security financing, and make available global and country-level research and analysis to improve coordination of the policy and financial response to the crisis.
The Bank hosts a Multi-Donor Trust Fund, Food Systems 2030 , that helps countries build better food systems, fostering healthy people, a healthy planet and healthy economies. The Trust Fund aims to deliver improved livelihoods and affordable, and nutritious diets for all, and progress towards the Sustainable Development Goals of zero poverty and hunger by 2030 and the climate goals of the Paris Agreement. Food Systems 2030 provides advice and analytical products to underpin policy options, funds to pilot innovative approaches, and information to build support for change in different country contexts. It engages with the private sector by supporting the design, piloting and de-risking of innovative public-private partnerships that advance development and climate goals.
The Global Agriculture and Food Security Program , a multilateral financing platform, is dedicated to improving food and nutrition security worldwide. Launched by the G20 in the wake of the global response to the 2007–08 food price crisis, GAFSP works to build sustainable and resilient agriculture and food systems in the world’s poorest and most vulnerable countries. Since its inception in 2010, the Program has mobilized more than US$2 billion in donor funds to reach more than 16.6 million people. GAFSP provides financial and technical resources – investment grants, technical assistance, concessional finance, and advisory services – to demand-driven projects along the food chain to accelerate the transformation of food systems at scale.
The World Bank leads the Food Systems, Land use and Restoration Global Platform (FOLUR) , financed by the Global Environment Facility, in partnership with UNDP, the UN Food and Agriculture Organization (FAO), the Global Landscapes Forum and the Food and Land-use Coalition. FOLUR is a $345 million, seven-year program that aims to improve the health and sustainability of landscapes that produce the world’s food. FOLUR targets sustainable production landscapes in 27 country projects for eight major commodities (livestock, cocoa, coffee, maize, palm oil, rice, soy, and wheat).
The World Bank chairs the System Council of CGIAR , a global partnership that advances cutting-edge science to reduce rural poverty, increase food security, improve human health and nutrition, and ensure sustainable management of natural resources.
For more information, contact Clare Murphy-McGreevey on [email protected].
Last Updated: Sep 19, 2023
AROUND THE BANK GROUP
Find out what the Bank Group's branches are doing on Agriculture.

STAY CONNECTED
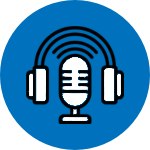
Programs and Trust Funds
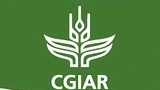
CGIAR Global Agricultural Research
CGIAR advances cutting-edge science to reduce rural poverty, increase food security, improve human health and nutrition, and ensure the sustainable management of natural resources.
Food Systems 2030
Food Systems 2030 is an Umbrella Multi-Donor Trust Fund that helps countries build better food systems by 2030. Food Systems 2030 helps countries rethink and transform their food systems from farm to fork, progressing ...
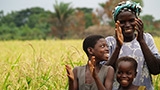
Global Agriculture and Food Security Program
The Global Agriculture and Food Security Program (GAFSP) finances investments that increase incomes and improve food and nutrition security in developing countries.
Additional Resources
Media inquiries.
This site uses cookies to optimize functionality and give you the best possible experience. If you continue to navigate this website beyond this page, cookies will be placed on your browser. To learn more about cookies, click here .
Search Issues
Vol. XXXVI, No. 3, Spring 2020
How to Improve the Social Benefits of Agricultural Research
By Marci Baranski , Mary Ollenburger
The Green Revolution left millions of the world’s smallholder farmers behind. Increasing the role of farmers in agricultural innovation can help them catch up—and will lead to better science in the process.
Famines have killed tens of millions of people across the centuries. But as the global population rose from 2.5 billion people in 1950 to nearly eight billion today, mortality from famine was radically curtailed. What accounted for this remarkable accomplishment? Perhaps the most commonly cited factor is the Green Revolution, typically summarized, as in this online encyclopedia entry , as the “ great increase in production of food grains (especially wheat and rice) that resulted in large part from the introduction into developing countries of new, high-yielding varieties, beginning in the mid-20th century.”
Today, many efforts to address rural poverty and food insecurity in low-income nations through agricultural research for development (AR4D) programs are built on this narrow view of the Green Revolution. And indeed, the accomplishments of the Green Revolution should be celebrated. But they have also been misunderstood. To start, the contribution of Green Revolution crop varieties to preventing famine is overstated. For example, India’s food production was increasing steadily more than 15 years before Indian farmers started adopting Green Revolution wheat in 1967. The Green Revolution’s successes were also concentrated among wealthier farmers. This was in part because research organizations directly targeted “successful” farmers to test new technologies, with the idea that best practices would trickle down to poorer farmers. But this often didn’t happen. Poor, smallholder farmers often lacked access to credit, could not afford inputs such as fertilizers, or grew different crops than the ones developed by Green Revolution research. Overall, the Green Revolution did little to reduce food insecurity and actually increased rural inequality, leaving behind the poorest and most vulnerable.
The continued failure of AR4D donors and research organizations to fully understand these ambiguous and uneven outcomes of the Green Revolution is leading to simplistic scientific and policy approaches that can further exacerbate economic and social inequities experienced by millions of smallholder farmers worldwide. Accordingly, we argue that agricultural research policies need to accommodate the complexity of the real world of smallholder farming if they are to achieve their dual goals of enhanced food security and poverty relief.
Modernizing the peasantry
Starting in the 1940s, the United States became involved in funding international agricultural research centers through the Rockefeller Foundation, the Ford Foundation, and later the US Agency for International Development (USAID). This investment in international scientific infrastructure, along with the spread of synthetic fertilizers, led to a growth in production of grains such as wheat and rice. This era was later dubbed the “Green Revolution.” Orchestrators of the Green Revolution focused on maximizing yields in targeted areas in order to increase aggregate food production. The Green Revolution also promoted commercialization of agriculture, which fit with America’s political goal of modernizing the global peasantry to promote democracy and economic growth. Production of maize, wheat, and rice increased in the areas targeted by the US-based organizations, and new “packages” of modern crop varieties, fertilizers, and irrigation were applied in geographically disparate regions, especially for wheat. Under certain conditions (typically those of larger farms), farmers increased their yields and incomes. Although the Green Revolution coincided with poverty reduction in parts of the world, a multitude of political, economic, and infrastructural changes were also occurring, and academic scholars continue to debate the extent to which agricultural innovation was responsible for reduced poverty.
A key principle of the Green Revolution was that agricultural science could produce universally applicable agricultural technologies. Donors would fund international research centers to develop universal technologies, tested in controlled field trials. National centers and extension services would adapt those technologies to local conditions where needed, but as much as possible farmers’ fields would be expected to imitate the research fields, which were typically well maintained with adequate irrigation, drainage, fertilizers, and pest control. Deviation from these conditions was seen by researchers as evidence of inadequate agricultural practices.
The commonly accepted story that Green Revolution technologies prevented global famine and reduced poverty by modernizing global agriculture continues to dominate the imagination of the public, scientists, and donors alike. Central to this story is a simplistic, linear view of agricultural innovation itself: scientists conduct controlled research in the lab or field, then publish or patent the results, which are then picked up by industry or government and made available to farmers, who benefit from improved crop varieties, greater productivity, and so on.
This linear story is exemplified by the most famous innovation of the Green Revolution: broadly adapted wheat. The scientist Norman Borlaug , while working with the Rockefeller Foundation in Mexico, realized that certain tropical spring wheats could be grown over multiple agroecological zones. Borlaug, who later won the Nobel Peace Prize, bred several broadly adapted wheat varieties that were successfully grown in Central and South America, India, Pakistan, and parts of Africa and the Middle East. Yet other key crops—maize, winter wheat, and rice—had much more limited success with broad adaptation. Even wheat was broadly adapted only in the sense that it yielded well under well-fertilized, irrigated conditions regardless of latitude. Further, the adoption of Borlaug’s wheats in India displaced the production of more nutritious legume crops and did little to reduce widespread malnutrition or poverty. Nor did the new wheat varieties automatically find their way into farmers’ fields. Coordinated political and infrastructural changes, such as expanded agricultural extension services, were necessary to facilitate rapid transfer of technology in certain parts of the world, while investments in irrigation and fertilizer were necessary to assure improved crop yields.
In the decades following the introduction of Green Revolution technologies, numerous studies made it clear that new agricultural technologies had mostly benefited wealthier farmers with large landholdings who had access to irrigation, fertilizers, and markets. But small farms were, and today remain, an important part of the food system, the economy, and the social organization of much of the world. Worldwide, 80% of farms are smaller than two hectares (five acres). In low- and lower-middle income nations, these account for up to 40% of total agricultural land; farms smaller than five hectares make up about 90% of the agricultural land. In India today, agriculture accounts for 25% of the nation’s gross domestic product and 60% of employment; in sub-Saharan Africa the numbers are higher still. And in many areas of the world, the average size of farms is actually decreasing.
Science for the real world
The linear view of agricultural science and innovation that underlay the Green Revolution virtually guaranteed that smallholder farmers could not benefit from it. For a technology to be adopted by smallholders, it must perform in the real-world conditions that they confront. Such conditions include labor shortages and uncertain access to fertilizer and other inputs—either because they are not available in local markets or because of a lack of capital or access to credit. In order to design technologies that lend themselves to adoption by smallholders, those technologies must be tested with the involvement of the farmers, and on their land. This type of on-farm, participatory experiment is, however, difficult to analyze and interpret because of the high variability of conditions on farmers’ fields. In contrast to the uniform, professionally managed fields found on research stations, farmers’ fields may have trees, termite mounds, or slopes that result in variability at very small spatial scales. Weeds and insects may not be controlled equally well by all farmers testing a new seed variety or soil fertility practice, confounding efforts to control variability. Soil physical characteristics can range from sand to clay over areas of only a few hectares. Soil fertility is also influenced by the history of cultivation on a plot—long periods of continuous cultivation can lead to depletion of organic matter and acidified soil, which both decrease the soil’s fertility and make standard fertilization practices less effective.
Agricultural science that can contribute decisively to the livelihoods of smallholder farmers must accommodate this variability and include actual farms—not just highly controlled test fields—as sites for research. Yet because it is impossible to account for all the factors that can contribute to yield variability, some scientists dismiss on-farm research as insufficiently rigorous. But if science is supposed to provide reliable knowledge about the real world, then agricultural research must study the highly variable conditions in which smallholder farmers work. A study of two thousand soybean trials conducted on farms in 10 sub-Saharan African countries as part of the N2Africa project showed that yield variability at the field level was higher than variability among countries or years. This level of local variability makes adoption of new crop technologies riskier for farmers.
Technologies that lend themselves to adoption by smallholders must be tested with the involvement of the farmers, and on their land.
Understanding the reasons why one farmer’s yield was high while a neighbor’s was low can reduce the uncertainty, and the risk, of technology adoption. For example, a 2018 study of 372 on-farm trials carried out in the West African country of Mali demonstrated that yields from a range of new technologies were significantly influenced by farmer-identified soil type and by the previous crop grown in the field where the technology was tested. This type of information, which is inherently place-based, allowed researchers and farmers to develop multiyear plans that would place new crop varieties and management practices in the locations and sequences where they have the best chance to succeed. This in turn gives farmers greater confidence in unfamiliar farming practices, and increases the likelihood that they will adopt such practices.
In addition, smallholder farmers usually have multiple sources of income both within and beyond the farm. Off-farm work can include wage labor for better-off farmers, seasonal or long-term migration, small-scale commerce, or trades such as carpentry or basket-making. Agricultural innovations that require additional investments in labor or capital must not only improve on existing farming practices but also compete with these other investment opportunities. Smallholder farmers might reasonably decide that the costs of adopting agricultural innovations that might work well on larger farms outweigh the benefits. In many areas, even the best agricultural technologies are not, on their own, capable of lifting smallholder farmers and their families out of poverty.
Where the money goes
Major US-based donors and organizations working to advance agricultural research for development continue to embrace a linear model that focuses mostly on technological solutions to increase crop yields, with insufficient consideration of the socioeconomic complexity involved in advancing food security or poverty reduction.
One of the major players in the AR4D space is the Bill & Melinda Gates Foundation, which since 2006 has donated roughly $5 billion to agricultural development in sub-Saharan Africa and South Asia with the goal of “agricultural transformation” that is equitable and brings low-income households out of poverty. But the foundation falls into several traps of the linear model of technology development, including setting its strategies with little to no involvement from farmers, focusing on technology dissemination and increasing farmers’ participation in formal markets to “achieve impact,” and emphasizing the “scalability” of agricultural technologies across disparate contexts. The foundation also cofounded the Alliance for a Green Revolution in Africa, which seeks to advance agricultural development in sub-Saharan Africa. Yet the foundation’s strategies do not address why the first Green Revolution failed to take hold in sub-Saharan Africa. Although the foundation acknowledges that Green Revolution technologies’ reliance on fertilizer and irrigation has been a barrier to their adoption on the continent, and that crop varieties must be adapted to fit African environments, its approach to research and development remains technology-driven, with a focus on broadly adapted crop varieties. This approach fails to recognize that improving staple crop production has rarely lifted anyone out of poverty.
The US government is another major AR4D investor. Its Feed the Future Initiative, started under the Obama administration and led by USAID, has spent over $3.5 billion on agricultural development since 2010. The initiative focuses on technology transfer, scalability, and demonstrating impact on short time scales. Feed the Future documents show a commitment to the linear model of technology development, but also emphasize collecting “ongoing, iterative feedback from end-users, stakeholders, and technology scaling partners to inform activities throughout the research pipeline.” In practice, however, participation is often limited to token stakeholder groups whose inputs to the process come too late to meaningfully influence research directions. The websites of the 24 Feed the Future Innovation Labs demonstrate an overwhelming focus on basic and applied research for crop varietal improvement, increased productivity with reduced environmental impact (“sustainable intensification”), and increased involvement in formal markets, with little to no mention of agroecological context or end-user needs. For example, several of these labs aim to develop heat-tolerant crop varieties. Despite language about specifically targeting smallholder farmers and women, the first adopters of innovations such as heat-tolerant varieties will almost certainly be farmers of higher socioeconomic status. Feed the Future also promotes the assumption that farmers should continue to grow staple grain crops, rather than explore alternative income sources within or out of agriculture. Narrowly focusing on crop improvement locks farmers into a production system rather than expanding options for addressing systemic economic and environmental issues.
Distorted incentives
Implementation of AR4D is substantially carried out by an international research network funded by national, international, and private donors. Known as CGIAR, the network emerged during the Green Revolution and continues to promote centralized agricultural research for development through the creation of what it terms “global public goods,” such as new crop varieties. CGIAR receives major support from both the Feed the Future project and the Gates Foundation; for example, nearly 40% of its wheat and maize research spending comes from these two sources.
Starting in the 1980s, international aid began focusing on measuring “impact,” and CGIAR typically did this through studies of successful technological change. Adoption studies provided the evidence for justifying further investments in AR4D. But we are troubled by the widespread reliance on farmer adoption studies to provide evidence of impact. These studies typically count the number of farmers or acres that adopt a new technology, but do not look at differences among socioeconomic subgroups, or define “adoption” in a way that is generalizable. A farmer who is reported to have adopted a new groundnut variety may have planted a 10-meter by 10-meter trial supervised by researchers, may have planted half an hectare of seed provided by the project, or may have simply purchased seed. Moreover, rejection of a new technology is rarely considered, so if farmers return to their original groundnut variety in the next season, they may still be included in the project’s total number of farmers adopting improved varieties. When adoption is measured as part of a specific project, farmers often receive support including training, free or subsidized fertilizer or other inputs, or a guaranteed buyer for their crop.
Despite language about specifically targeting smallholder farmers and women, the first adopters of innovations such as heat-tolerant varieties will almost certainly be farmers of higher socioeconomic status.
Quantitative measures of impact are important considerations when evaluating AR4D. But adoption and impact are not the same. Projects with the long-term objectives of decreasing rural poverty may use adoption as an intermediate metric, but the connection between adoption of new technology and impact on farmer incomes and livelihoods is difficult to demonstrate. Using adoption to evaluate impact reinforces the view that farmers are mere passive recipients in the innovation process. It strongly biases AR4D toward linear approaches that view research as best carried out under controlled conditions at the beginning of the innovation process. It thus fails to recognize the value of iterative processes where farmers provide feedback to scientists that can allow product improvement over time, to say nothing of including farmers’ input in the development of research questions.
Agricultural research is slow. Field trials can be conducted once, maybe twice a year. Interannual climate variability makes multiyear trials important for evaluating new technologies. Building the relationships and level of trust needed for collaboration among multiple stakeholders—farmers’ organizations, extension services, national and international research centers, and universities—also takes time. But CGIAR researchers typically rely on short-term project-based funding to cover operational and administrative costs as well as their own salaries. Projects are often funded on three-to-five-year time frames, and evaluated on an annual basis. Because researchers are directly accountable to donors, projects that allow short-term, quantitative demonstrations of impact are incentivized. Accountability to the intended users of the technologies is in turn disincentivized. One result is that technologies that may appear to have widespread success during the course of a project are abandoned once the project ends and the incentives it provided disappear. Paradoxically, increased emphasis on quantifying impact may actually reduce the long-term impact of a project.
Whose excellence?
CGIAR is also organized around regional research centers that focus on particular crops. The International Maize and Wheat Improvement Center (CIMMYT) in Mexico is responsible for maize and wheat worldwide; the International Crops Research Institute for the Semi-Arid Tropics (ICRISAT) in India researches sorghum, millet, and groundnut, among other crops; and the International Rice Research Institute (IRRI) in the Philippines researches rice, except for African rice varieties, which are the purview of AfricaRICE. Each of these centers has regional subcenters and satellite locations around the world, all focused on the center’s mandated crops. A center’s focus on particular commodity crops limits the flexibility of researchers—if farmers are interested in rice, but researchers at a nearby location work for ICRISAT, there is no way for those researchers to meet farmers’ needs. Attempts have been made to develop partnerships and structures that allowed multiple stakeholders to participate in the research process. However, despite their increased complexity these collaborative programs were still expected to demonstrate impact in terms of quantitative metrics, such as adoption, on an annual basis.
CGIAR currently promotes three “research support” platforms: Big Data, Excellence in Breeding (plant breeding using “cutting edge” tools), and Genebank (maintaining diverse seed collections and facilitating their use in breeding as a source of desired traits). Notably absent is any support for on-farm research, systems analysis, or technology development processes that include farmers’ and other stakeholders’ input. Rather, the focus is on better data analytics, crop modeling, and other computational methods that reinforce CGIAR centers’ traditional focus on developing improved commodity crop varieties. If “excellence in breeding” research is defined as computationally intensive, high-throughput, high-tech studies carried out in controlled settings, then on-farm research and participatory plant breeding are not “excellent.” Instead, these are seen as separate, lesser activities.
Donors and researchers alike often view breeding and varietal development as the principal role of agricultural research. But better seeds don’t solve all of farmers’ problems. A high-yielding rice variety may not be adopted if it cannot be hand-threshed and machine threshing is not available locally. A sorghum hybrid that produces high yields a few weeks before the local varieties may sound ideal, but if all the birds in the area show up for an early feast, the farmer may harvest almost nothing. In many cases, low-technology agronomic changes in crop patterns and simple technologies such as nets and stakes may contribute as much or more to increasing farmers’ yields and incomes than the latest crop variety. But broadly adapted, commodity plant breeding to produce global public goods has always been the primary focus of CGIAR, and so it remains.
What’s the problem?
The dominant approach to AR4D does result in new technologies, but many of the technologies remain on the shelf rather than becoming integrated into farmers’ production systems, especially for smallholder farmers in low- and low-to-middle-income countries. As we have emphasized, the linear approach to AR4D has thus failed to provide adequate benefits for smallholder farms, despite their importance in many regions, especially in South Asia and sub-Saharan Africa. Part of the problem, in our view, is that government programs, donors, and scientists alike continue to view farmers as passive recipients of their technological benevolence. But looking at smallholder farmers as knowledgeable customers, and at agricultural technologies as products engineered to meet customer need, suggests an alternative “product development” model of innovation that is more likely to produce relevant, usable technologies to improve food security and reduce poverty.
The central idea behind product development is to increase the chance of product commercialization. Product development can be described as a series of stages encompassing problem definition, concept development, design, prototyping, final product development, and commercialization, but each stage is iterative and includes multiple sources of input. The starting point in a product-development approach to AR4D is to engage clients at the beginning of the research process. In many projects, the problem is defined as poverty and food insecurity. If we take that as our starting point, we then move to concept development: what are the ways we can address the problem? In the linear approach, a crop researcher might assume the best way to improve a client’s income and food security is through increasing the yields of the crops the client grows (or should grow). However, engaging with the client reveals a tableau of their needs, constraints, and priorities. For example, if a farmer’s goal is to increase income, off-farm employment might be a better pathway for improving household income than adopting high-yielding varieties. Even where, for example, an improved variety is chosen as a means to address the problem, the process does not revert to a linear model.
Within an iterative process of product development, prototypes are tested under a variety of conditions (including by clients) and the product is altered based on the findings to better address the conditions of actual use. Users are part of the innovation process. Moreover, understanding the economic and social implications of the product’s introduction is critical to its success in solving the problem. In the linear approach to agricultural research, new technologies are usually evaluated by only a few factors—yield improvements, disease resistance, and maybe cost. But many more factors go into a client’s decision to use a technology. For example, what are the alternatives and how is this product different? Is the market for selling this product well developed? Does the product exacerbate gender inequity by differentially increasing women’s labor over men’s? These questions are made explicit through the product development process. In contrast, under the current system of research (with its focus on short-term adoption), consideration of user needs and socioeconomic factors conflicts with the researchers’ incentives.
Fine, but would you want to eat it?
An example of how AR4D research can approach crop breeding through a product-development approach is a program in Mali developed by Eva and Fred Weltzien-Rattunde, both agricultural scientists with extensive plant breeding experience in tropical and subtropical settings. The couple, working for ICRISAT, ran a sorghum breeding program that develops locally adapted sorghum cultivars and hybrids through extensive work with farmers and helps make those varieties widely available by working with farmers’ seed cooperatives. This process includes farmers from the beginning. Some of these farmers plant diverse seed mixtures representing a wide range of plant traits. They then participate in the selection of desirable traits from that population, from which new varieties may eventually be developed. As the breeding process continues, researchers hold culinary test days in villages, where local women process and prepare the local staple, tô, from several sorghum varieties in development. Throughout the preparation process, measurements are taken to quantify processing losses, and qualitative information is collected from the women doing the processing. At the end, men, women, and children conduct taste tests and rank the varieties on flavor, texture, appearance, and any other characteristics they think are important. Finally, ICRISAT works with seed cooperatives to train farmers in producing certified seed of specific varieties and hybrids, and crucially also in accounting, organizational, and management skills. Mini-packets of seed are sold by the cooperatives and through ICRISAT projects, and follow-up research on the buyers of the mini-packets has provided information not only on adoption but also on how these improved varieties spread through both formal and informal seed systems.
Government programs, donors, and scientists alike continue to view farmers as passive recipients of their technological benevolence.
The continuous involvement of farmers at multiple stages of the research process contrasts markedly with standard AR4D approaches, as used at the Pan-African Variety Trial, part of the USAID Feed the Future program’s Soybean Innovation Lab at the University of Illinois. There, varieties developed and released in the United States and Brazil, and by CGIAR centers and private partners in Africa, are tested in strictly controlled trials, and only the best varieties from those trials are tested on farmers’ fields. Farmers’ feedback into this breeding system is minimal at best. As the project claims, this may well be a way to quickly get improved germplasm into farmers’ hands. But it also faithfully replicates the linear model of the first Green Revolution, and thus is likely to replicate the weaknesses that compromised that approach.
We are contrasting these programs to emphasize that participatory and place-based research can produce the types of widely used technologies—public goods, in AR4D parlance—that are promised by standard Green Revolution approaches. The difference is that the resulting products may be applicable to a much more diverse array of publics—not just wealthier farmers but smallholder farmers in diverse agricultural and socioeconomic settings. Technologies need adequate experimentation and adaptation in different social and agroecological conditions to derive insights about their successful application. Identifying the socio-ecological niche for which a new technology is appropriate can aid efforts to disseminate the technology in other places with similar conditions. For example, technologies such as intercropping that maximize the efficiency of land use may be appropriate for areas with high population density and limited farm size, while labor-saving technologies such as herbicide use may be more attractive in areas with low population density, where farm size is defined by how much area a farm household can plant and maintain. Grain legumes that are successful in well-drained soils will be of little use to a poor farmer whose land sits in an often-flooded depression. Coordinated participatory research in a range of socio-ecological niches can be synthesized to compare findings from multiple locations. And localized, participatory research can still produce technologies that are broadly adapted.
Farmers are innovators
Donor agencies may be starting to recognize the need to move AR4D closer to a product-development approach. A 2017 USAID report on scaling up agricultural technologies noted that examples of successful commercialization “are neither those where research organizations drive the entire scaling process nor those involving sudden handoff from research organizations to commercial actors, with no further research involvement. The ‘handoff’ model makes insufficient allowance for the need to modify and adapt technologies iteratively in response to market responses.” Successful product development requires integration of multiple stakeholders—such as social scientists, engineers, extension workers, traders or exporters, and farmers—who traditionally have not been involved in agricultural research until the product is already considered “done.”
But words are not sufficient. Within the international and national research systems, incentives must be changed to encourage product-development approaches to research that are place-based and participatory. If individual scientists are evaluated based primarily on the number of journal articles they produce or the rapidity with which they can develop new crop varieties, they have no incentive—in fact, given the time required, they have a fairly strong disincentive—to work in an iterative development process. Scientists should instead be given flexibility to champion promising technologies through the development process, participating in the ways that advance that process, rather than solely focusing on advancing a research agenda, with any benefit to the client a secondary concern at best. Our research in India found that scientists wanted to work more with farmers but lacked permission from their employers, resources, or incentives to do so.
Despite decades of efforts to promote change, agricultural research for development continues to be dominated by a linear model that places scientists at one end and farmers at the other. Linear research processes are ill-suited to tackling the problems of food security and poverty reduction that are the stated justification for this billion-dollar research endeavor, but the structure and incentives of research institutions reinforce the linear model. A shift in perspective that treats agricultural technologies as products being designed for clients, instead of “best practices” determined for passive or idealized beneficiaries, would promote a more inclusive process leading to technologies better suited to address the complex socioeconomic problems at which they are aimed.
Implicit in this framing is the idea that scientists do not have a monopoly on innovation. Problem-solving is a collaborative process wherein ideas come from a range of sources: farmers, traders, extension offices, sociologists, and yes, agronomists and crop breeders. A product-development framework accepts the possibility that an identified problem, such as poverty or food security, might be best addressed with new agricultural technologies—but it might not. The avenues pursued might align with scientists’ research agendas, or, again, they might not. It is not only scientists whose attitudes should change, but also the donors and research administrators across the AR4D landscape. The flexibility and uncertainty of a product-development process precludes narrowly defined objectives, and turns carefully constructed work plans associated with linear innovation models into implausible fictions. The ways success and failure are defined and measured must shift, requiring institutional innovation on the part of donors, research institutions, and the broader community of agricultural scientists.
This level of necessary disruption may seem impossible—and it may be impossible. But 70 years after the ideals of the Green Revolution began to take shape, a continued focus on high-tech solutions to systemic problems only exacerbates economic inequality, disempowers smallholder farmers, and restricts the range of possibilities considered for addressing the needs of millions of people. In a world of increasing food demand, increasing inequality, and changing climate, the challenges farmers face are too important to be held hostage by outmoded beliefs about the best ways to connect science and innovation to human betterment.
Join the Conversation
Sign up for the Issues in Science and Technology newsletter to get the latest policy insights delivered direct to your inbox. When you do, you'll receive a special offer for nearly 50% off a one-year subscription to the magazine—or simply subscribe now at this special rate .
- Name * First Last
- Comments This field is for validation purposes and should be left unchanged.
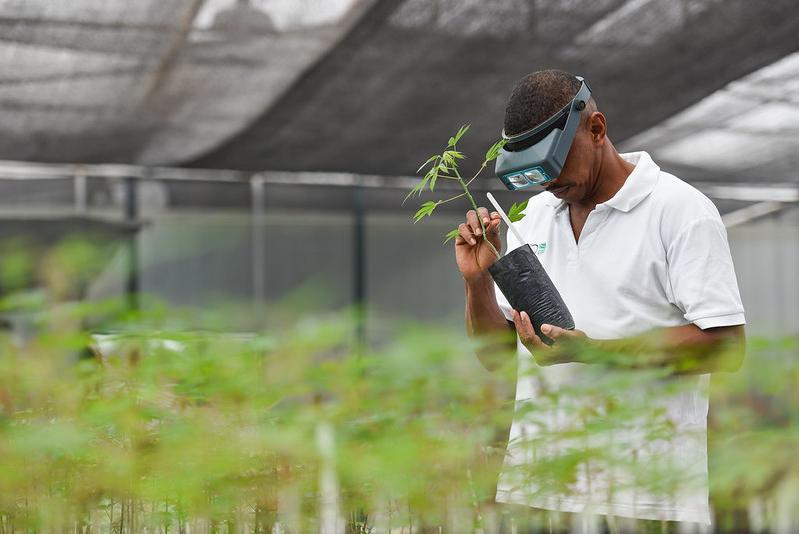
Celebrating Science & Innovation in Agriculture
A story by:.
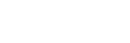
Agriculture today is about so much more than a farmer simply planting a seed, rearing a cow or catching a fish. It takes a whole ecosystem and a host of actors to work together to produce the food we need for a population of more than eight billion people.
This complex agricultural production system has evolved over time through scientific discoveries and other innovations. It is this dynamic nature that will equip agriculture to cope with the competing challenges of addressing food and nutrition security, improving livelihoods, combatting climate change and sustainably managing natural resources.
Let’s take a closer look at “science and innovation” in agriculture: the ways it works, the benefits it provides and the future challenges it must still help us to overcome.
Natural Resource Management
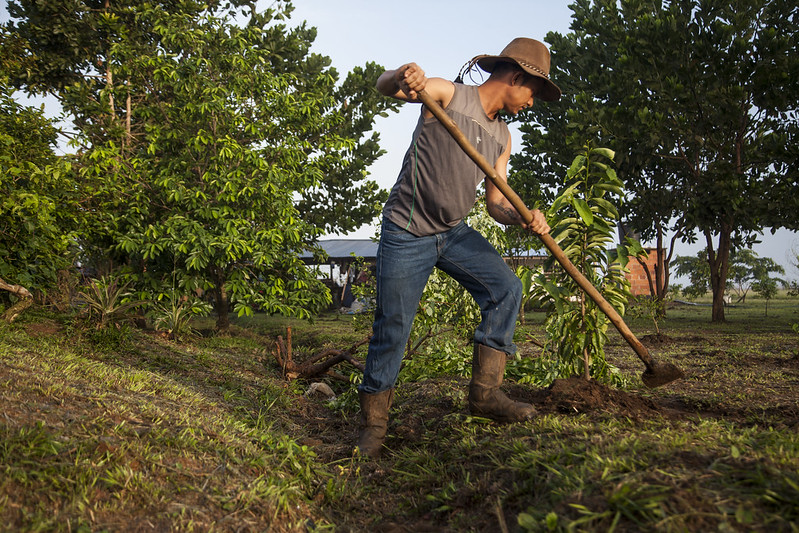
The world’s 570 million farmers are arguably the most important stewards of the earth’s land, water and biodiversity. Worldwide, farming uses around 40% of total land area , two-thirds of water withdrawals and 85% of water consumption today. This is up from around 7% of total land area back in the year 1700 when the population was less than 10% of what it is today.
Advances in technology and farming practices have helped farmers become much more productive, growing crops efficiently in areas most suitable for agricultural production.
Without these advances, far more land would need to be cultivated to produce the food we need today. For instance, it has been estimated that we could produce the same amount of total food grown fifty years ago on less than one-third the amount of land used back then. If yields had stayed the same since 1961, we’d need to cultivate more than double the amount of land to feed the population today – a shift from 12.2 billion acres to at least 26.3 billion acres. That’s 82% of our total land area on earth.
Similarly, farmers tend to use water more efficiently as their yields increase. According to the International Water Management Institute , a farmer who grows about eight times the yield of another farmer uses only about three times as much water to do so.
In the coastal region of southern Bangladesh, soil salinity and a shortage of water for irrigation typically keep farmers from growing a crop in the dry season. However, a group of innovative women farmers is increasing production of maize, wheat and mung bean during the dry season despite these challenges. Key to their success has been using simple machinery to reduce tillage. This allows for earlier planting and keep crop residues on the soil surface to conserve soil moisture and reduce salinity. The women have also used crop varieties that mature faster.
In central Bangladesh, where the cost of irrigation and farm labour is skyrocketing, farmers and local service providers are teaming up to plant wheat, maize and legumes on raised beds to reduce labour and water requirements.
The International Maize and Wheat Improvement Center (CIMMYT) and the Cereal Systems Initiative for South Asia in Bangladesh (CSISA-BD) are working in partnership with the Regional Wheat Research Consortium of the Bangladesh Agricultural Research Institute on this initiative.
Indonesia’s rich landscape makes it ideal for cultivating commodities like palm oil. Yet the increasing incidence of farmers burning land to bring it into production is having grave environmental consequences. Satellite-mapping company DigitalGlobe is working with the World Resources Institute in Indonesia to create a better picture of the earth’s surface, as part of the “Global Forest Watch” (GFW) initiative. Global Forest Watch is an interactive online forest monitoring and alert system designed to empower people everywhere with the information they need to better manage and conserve forest landscapes.
DigitalGlobe’s technology in Indonesia enables the team to see high-resolution visuals of fires and haze patterns that are affecting the environment. The images are then passed on to government agencies who are then better equipped to locate those responsible and develop better policies to prevent this from happening. Global Forest Watch allows users to create custom maps, analyse forest trends, subscribe to alerts, or download data for their local area or the entire world. Users can also contribute to GFW by sharing data and stories from the ground via GFW’s crowdsourcing tools, blogs, and discussion groups.
The low-rainfall area of Barmer, Rajasthan, India can remain dry for up to 11 months of the year. If the rains do not come, farmers struggle to find enough water for their food crops, or for the goats that families keep as a source of milk and manure. Many men have also migrated to the city to find work.
The International Crops Research Institute for the Semi Arid Tropics (ICRISAT) is working with women in Barmer, offering interventions to help reduce the drudgery of the labour women must undertake to survive. Women are helped to organise themselves into self help groups, and taught how to harvest rainwater. Using this harvested water, the women are taught how to keep small agri-horticultural gardens, which they can also use to earn an income. Improved seeds of pearl millet and other beans are also provided.
One farmer who has seen great success is Mani Devi. She used the profits from her garden to buy a sewing machine, and is now training women in her family and the rest of the village on how to use it.
Drones, or unmanned aerial vehicles (UAVs), are most often linked to the military. However, potato scientists at the International Potato Centre (CIP) are putting them to another use – to gather data on plant life.
Remote sensing projects are helping scientists to observe how plant life develops and evolves across landscapes over time through characteristics such as biomass, nutrient content, disease and water use. In this sense, scientists can use UAVs to collect images and data on plant numbers and type, the lay of agricultural land, and how crops are being affected by disease and climate change.
Currently CIP uses a number of airplane and helicopter UAVs including an Oktokopter XL. This insect-like remote flying machine was acquired from MikroKopter (Germany) and assembled in CIP and is capable of carrying up to two kilograms of camera and computer equipment and flying at altitudes of over 100 metres for up to 12 minutes depending on the application. The Oktokopter XL is also able to fly at a stationary position, which makes it an excellent tool for aerial photography.
The forests of the Congo basin stretch over two million square kilometres, making it the second largest rainforest area in the world. Forests are essential for local and global life, as they not only provide food and a livelihood for the community, but also help prevent global warming by storing vast amounts of carbon from being released into the atmosphere.
But a rapidly growing population and a diminishing source of fish are leading people in the Democratic Republic of Congo to undertake slash and burn agriculture in the forest basin.
As part of a project run by the Center for International Forestry Research (CIFOR) and the United Nations Food and Agriculture Organization (FAO), a new course at the University of Kisangani is helping students collect better data on Congo’s forest, and perform agricultural activities whilst managing its biodiversity sustainably. There are currently few technically trained academics and scientists in DRC, and even fewer women involved in these subjects. Agents responsible for stewarding the forests are also attending courses, to learn more about the impact of human activity on forests.
In Matopo, Zimbabwe, conservation agriculture (CA) techniques have been proved to help farmers increase their yields and conserve natural resources .

Trained in CA, farmers use a variety of practices and technologies such as digging planting pits, improving soil fertility with manure, mulch or legumes, and precise planting. By multiple cropping and rotating maize with indigenous nutrient-rich crops, the soil quality builds over time. Crop residues trap moisture, control weeds, and maintain cooler soil temperatures.
Despite challenging climatic conditions over a period of 3 years, farmers reported increases in yields of sorghum, millet and maize, from an average of about 0.5 tonnes to between 3-4 tonnes per hectare.
Another survey in Zimbabwe compared CA with conventional farming practices under low, normal and high rainfall situations. Regardless of the level of rainfall, farmers achieved yields between 2 and 6 times of those under conventional practices whilst benefitting from reduced input requirements.
Agriculture for Impact has compiled a comprehensive collection of case studies of “sustainable intensification” in action.
Agricultural Extension
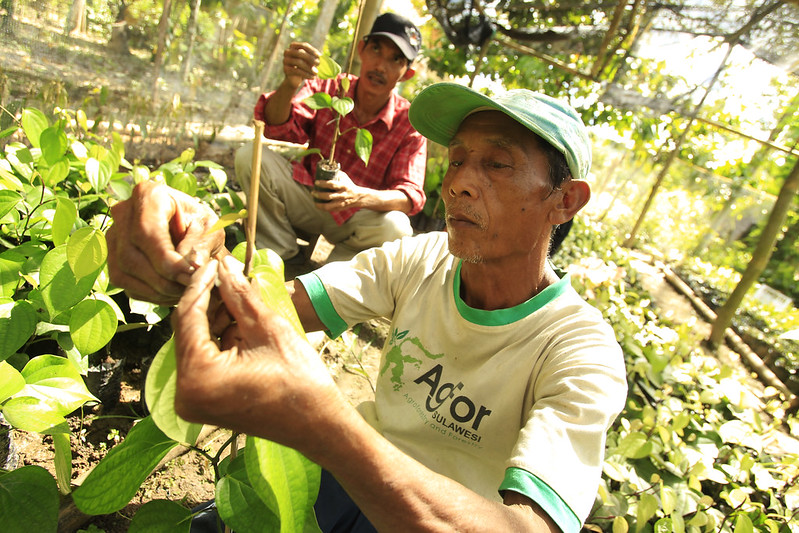
Innovation is not only driven by technological advances, but also through novel ways of organizing farmers and connecting them to the information they need.
Many smallholder farmers around the world still farm the same way their ancestors did thousands of years ago. Traditional farming approaches may continue to work for some, but new practices can help many to substantially improve yields, soil quality and natural capital as well as food and nutrition security.
For example, a smallholder farmer in Africa might still scatter her seeds across her land, rather than planting evenly and in rows. This stops the plant’s roots from taking up the maximum amount of nutrients from the soil. She might use seed saved from generation to generation. While indigenous seeds are important to protect genetic diversity, improved seeds could also help her to adapt to changing climate conditions, fight crop diseases and produce higher yields. She may plant the same crop year after year, rather than rotating her crops or planting a range of crops together to grow more, maintain soil health and diversify her family’s diet. And she might store her harvest in such a way that leaves it susceptible to pests, diseases and rot.
Sometimes, innovations to address these issues are taken to farms via extension training. Farmers themselves can be organized in innovative ways so they are reached more easily and effectively with information. The type and style of the extension itself has evolved much over time. For instance, advances in satellite mapping and information and communications technologies (ICTs) are transforming more traditional agricultural extension work today. Farming is becoming more precise and productive as a result.
Banana bunchy top disease (BBTD) is a devastating virus infecting bananas worldwide. It has had a huge impact on both industrial banana production and on subsistence farmers who depend on the crop to feed their families and provide income. Once established, it is very difficult to eradicate and manage the disease.
According to FAO statistics, Nigeria is the second largest banana producer in West Africa, contributing about 2.7 million tonnes annually. Together with partners, the International Institute for Tropical Agriculture (IITA) has launched the ‘Stop Bunchy Top’ campaign in Nigeria to help farmers fight the BBTD infestation.
Training focuses on how they can identify the disease and produce virus-free planting materials. It also creates awareness among extension workers and policymakers about the danger of BBTD and control measures, including the need to plant clean banana suckers to prevent their fields from becoming infested.
iShamba is a mobile based platform that enables smallholder farmers to access real time agricultural and market price information and expert advice via SMS and a call centre. Funded by the TradeMark East Africa’s Challenge Fund and devised by Mediae Company Kenya, iShamba complements Mediae’s existing Shamba Shape Up programme that uses reality TV to give farmers the tools and knowledge to improve profitability and productivity sustainably.
iShamba offers a free subscription service to farmers, giving them market prices for two crops in the two closest markets to them; a weekly weather forecast for their area, including likely rainfall and agronomy tip text messages aligned to the season in the farmer’s specific region in Kenya. This helps them to know exactly when to harvest their crops and which pests and diseases to be on the look out for.
Farmers are also currently benefiting from ‘special offers’ and ‘discounts’ from key East African Community-based agri-product suppliers who are keen to work with iShamba to reach new customers.
How can we measure which technologies will have the most impact on our food supply? Until now, policymakers have struggled to make informed decisions on how to boost productivity in their regions in the most sustainable way.
The recent report “Food Security in A World of Natural Resource Scarcity: The Role of Agricultural Technologies” compiled by the International Food Policy Research Institute (IFPRI) seeks to answer these questions.
The report reviews 11 agricultural technologies ranging from traditional low-tech practices to more advanced technologies, such as no-till agriculture, heat-tolerant varieties and rainwater harvesting. The report finds that different regions will need different technologies. For example, when the impact of drought tolerance is tested globally, it seems to have a low impact, as drought only affects some regions in some seasons and years. Combining multiple technologies (or ‘stacking’ them) can have an even greater impact. Adopting the three types of crop protection (against weeds, diseases and insects) together could reduce the number of food-insecure people by close to 9 per cent. An online tool has been developed to allow policymakers and researchers hands-on access to the results.
In 2013, CropLife Latin America formed a partnership with the United States Agency for International Development (USAID) to train Honduran farmers in good agricultural practices. The aim was to help lift 108,000 rural Hondurans out of extreme poverty by teaching farmers how to protect their crops from pests and disease.
AHSAFE-Honduras (the national member of CropLife Latin America) trained 120 USAID field officers on good agricultural practices and integrated pest management. The field officers in turn have trained more than 30,000 Honduran farmers. These farmers have been able to tackle pests and disease to improve the yield and quality of their crops and they are now earning higher incomes and enjoying a better quality of life. The project helped Emiliano Domínguez, a small-scale Honduran farmer, lift his family out of a life of poverty. He has been able to pay for a new house for his family of five and he has increased the amount of land he farms six times over.
The work in Honduras illustrates how public-private partnerships and good agricultural practices can address hunger and poverty around the world.
Rice production is not keeping up with demand in Africa. Changing diets, and rapid population growth mean that cultivation of this staple crop must dramatically increase its efficiency. To close the rice yield gap in Africa, AfricaRice, under the guidance of Dr. Kazuki Saito, has developed a decision support application (app) for providing African farmers with field-specific management guidelines called ‘RiceAdvice.’ It is an interactive tool, which generates recommendations based on farmers’ answers to around 20 questions.
RiceAdvice can identify the best choice of fertilizers to be purchased based on nutrient requirement and fertilizer prices, and their amounts and application timing. In RiceAdvice, farmers can also select their own target yield level based on their budget. It has been tested in the Senegal River valley and Kano, Nigeria. Results show that RiceAdvice guidelines give more than one tonne per hectare of yield advantage compared with farmers’ practices.
Saito is also leading a team that has developed the first version of a yield gap map for rice in nine African countries in the ‘Global Yield Gap Atlas’ website.
Seasonal rainfall forecasts can help farmers adapt to climate change and improve their resilience to climate shocks. The CGIAR Research Program on Climate Change, Agriculture and Food Security (CCAFS) is collaborating with the Senegalese National Meteorological Agency (ANACIM) to develop climate information services that are relevant to farmers on a broad scale. Farmers have been involved in every step of the process, helping meteorologists and other specialists package and communicate climate information.
As of August 2015, seasonal forecasts are transmitted nationwide through 82 rural community radio stations and SMS, potentially reaching 7.4 million rural people across Senegal.
Receiving climate information is one thing, but putting it into practice is another. In the beginning, some farmers were reluctant to join the project, as they were very accustomed to basing their actions only on their own know-how.
However, as the project was willing to integrate local knowledge into the climate information disseminated, these farmers became less resistant. Today, farmers are no longer content to wait for climate information, but go in search of it.
Often in Dalung, Ghana, the cold winter winds chase people inside in the evening. But when they have the chance to watch a television screen that teaches better ways to farm, a crowd of 200 villagers gathers in the thoroughfare. They lean in to hear a message that challenges all they know about rice farming and how to grow more than ever.

This is one of several ways IFDC’s Feed the Future Ghana Agriculture Technology Transfer project (ATT) reaches rural farmers through media-based extension. These methods inform farmers quickly and in a cost-effective way. In Dalung, farmers learned about new technology through public video screenings, held on mobile “video vans.” ATT focuses on producing and curating content that appeals to all demographics of farmers. The project helped produce a reality show, “Kuapa,” that promotes good agricultural practices and is aired on Ghana’s most popular TV network.
Elsewhere, the project collaborated with Farm Radio International (FRI) to host programs designed to benefit small-scale farmers. This program implements an Integrated Voice Response System to provide on-demand assistance to farmers who desire to learn more on their own time, and in their own language.
Together these initiatives are estimated to have reached more than 1 million smallholder farmers.
Improved Inputs
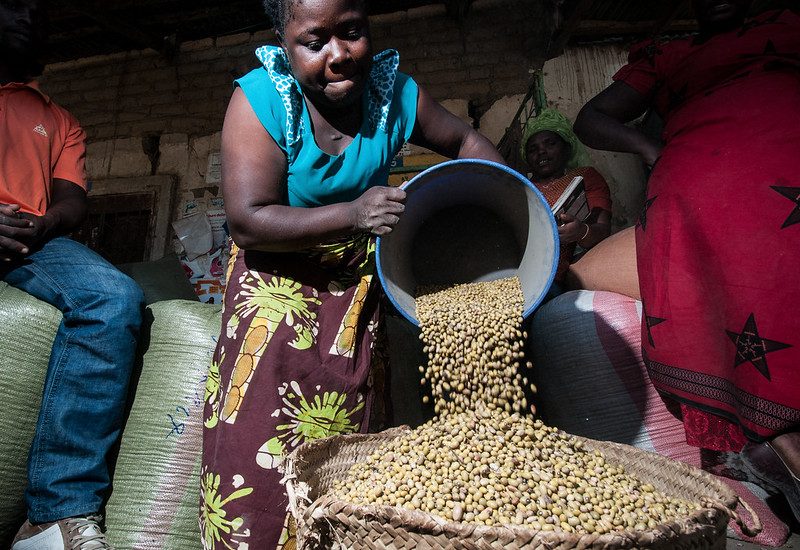
The quality, availability and proper use of agricultural inputs is at the heart of agricultural production and sustainability.
The crops that we grow today have been bred over the past ten thousand years to be quite distinct from their wild ancestors. Maize, for instance, has evolved from a species called teosinte, which is native to Mesoamerica. Similarly, modern wheat is the result of farmers in the Near East selecting for mutations which resulted from the natural crossing of different species of wild grass.

(Photo credits: John Doebley ; LaSalle )
Farmers today are faced with a changing climate, which demands seeds that can cope with increased incidents of droughts, heatwaves, floods and elevated salinity levels. This is happening while arable land per capita is ever decreasing, which compels farmers to maximize harvests on existing land.
To do this, the right inputs need to be used in the right amount and at the right time, in the right location. This is called the 4Rs, and is an integrated part of best management practices for improved and more efficient fertilizer application. For example, in more developed countries, global positioning systems (GPS) are helping farmers to track their use of fertilizer and match it very precisely to various soil types on their farm. It can also help them to identify potential pest or disease outbreaks.
Without pesticides and other pest controls, an estimated 70% of the world’s crop might be lost , rather than 42% today. This would require substantially more cropland being brought into production to make up for this loss.
Rice dies within days of being completely submerged, resulting in total crop loss. In Asia, where most of the world’s rice is grown, about 20 million hectares of rice land is prone to flooding. In India and Bangladesh alone, more than five million hectares of rice field are flooded during most of the planting seasons, which severely damages food supplies and farmer incomes.
In response, scientists have developed a “flood tolerant” rice variety that can withstand being submerged for two weeks. Scientists at the International Rice Research Institute (IRRI) scoured rice’s rich diversity for a gene that gives flood tolerance. After the gene (called SUB1) was found, it was bred conventionally into popularly grown rice varieties in rice-growing countries in Asia.
Several varieties with this “scuba” gene were released to India, Bangladesh, Philippines, Indonesia, Myanmar, Lao PDR, and Nepal. Farmer Nakanti Subbarao of Andhra Pradesh, India, was one of the first to adopt Swarna-Sub1 in his community. After seeing that he recovered 70 per cent of this rice after three weeks of flooding, he distributed Swarna-Sub1 seeds to his fellow farmers in Maruteru, which led to coverage of 800 ha in his village, and its nearby areas during the wet season of 2009.
Scuba rice is spreading fast in several countries over the last few years, and currently grown by more than five million farmers in Asia.
In Bangladesh about 60 per cent of the population eats fish at least every other day. Just as a nutritious diet is essential for our own healthy growth and development, the quality of feed given to farmed fish directly influences how fast and large they grow—in turn impacting the yield and farmer profits.
Yet it can be expensive and difficult to access quality feed. WorldFish, funded by the United States Agency for International Development (USAID) is working on the Aquaculture for Income and Nutrition (AIN) project in Bangladesh, training farmers to make their own fish feed from subsidized feed mills.
Since January 2014, AIN has established 62 feed mills and trained 430 farmers in feed production. Fish are now growing faster, and as growing feed is cheaper than buying it, fish farmers are enjoying a better income.
In a country where more than a third of the population lives below the poverty line, AIN is improving the productivity of household and commercial fish farms to help secure income and nutrition for rural farmers and their families.
In a country often referred to as the “pearl of Africa”, one crop—orange sweet potato (OSP)—has become a real gem for Ugandan farmers and their households. Bred conventionally through a process known as biofortification, OSP packs enough vitamin A to provide a child with a full daily dose. In Uganda, one-third of all children under five lack enough vitamin A, contributing to 29,000 deaths each year.
Diarrhoea is one of the leading causes of child mortality in Africa, but a recent study has shown that OSP can help children ward off or reduce the duration of the disease.
As their children enjoy the nutritional and health benefits of OSP, Ugandan farmers are realizing other gains from the crop, too; OSP is high yielding, early maturing, and drought tolerant, giving farmers good harvests and an additional way to make a living.
To date, nearly 300,000 Ugandan farming households are growing and consuming OSP in a project run by HarvestPlus. With demand for the crop continuing to rise, HarvestPlus and the Government of Uganda are working together to scale up nationally.
In 2005, a new strain of rust disease devastated lentil fields in Ethiopia. The local variety of seeds used by the farmers had little resistance to the new disease caused by unusual weather, a growing problem with climate change. Nearly 90 per cent of the farmers lost their produce.
In response, the Ethiopian government with the help of the International Center for Agricultural Research in the Dry Areas (ICARDA) stepped up efforts to improve legume varieties, with support from the International Fund for Agricultural Development and the government of Netherlands. ICARDA provided improved germplasm and varieties of lentils, chickpeas and faba beans for testing on farmers fields. The new varieties were first tested by the Ethiopian Institute of Agricultural Research (EIAR) for adaptability to the local environment, and after crossbreeding with local varieties, those with the highest yield potential were released.
Today, 20 per cent of Ethiopian farmers grow improved lentil varieties from ICARDA’s project, and legumes are now becoming popular. Apart from boosting yields, these crops are making soils healthier and reducing their expenses on fertilizers.
Legumes, being rich in protein and essential minerals such as zinc, also enrich the diets and nutrition of farmers and their families.
Both water and fertilizers play a critical role in agricultural production – in fact, each depends on the other. Fertilizer’s influence on yield depends on the water available to crops, and water’s impact on yield depends on nutrients’ availability to crops.

This presents a significant challenge for countries that have limited, or erratic rainfall, and/or poor access to fertilizers. Traditionally, approaches to boost production in dry regions have focused on individual interventions such as fertilizer use, or water conservation measures. But scientific trials have discovered that approaches that integrate both fertilizer and water use are much more effective.
For example, in the Tadla region of Morocco, laser-assisted land levelling, that reduces water runoff after rainfall, has resulted in both saving 20 per cent more water, and increasing crop yields by 30 per cent. Tiered ridges that capture rainwater have a similar effect: sorghum grain yields at on-farm locations in Burkina Faso were higher with the combination of fertilizer and tied ridges than with either fertilizer or tied ridges alone.
Agronomists at the International Fertilizer Industry Association, together with partners, have produced a scientific book that reviews the latest knowledge on plant nutrition and water management that can optimize water productivity and fertilizer use efficiency and effectiveness.
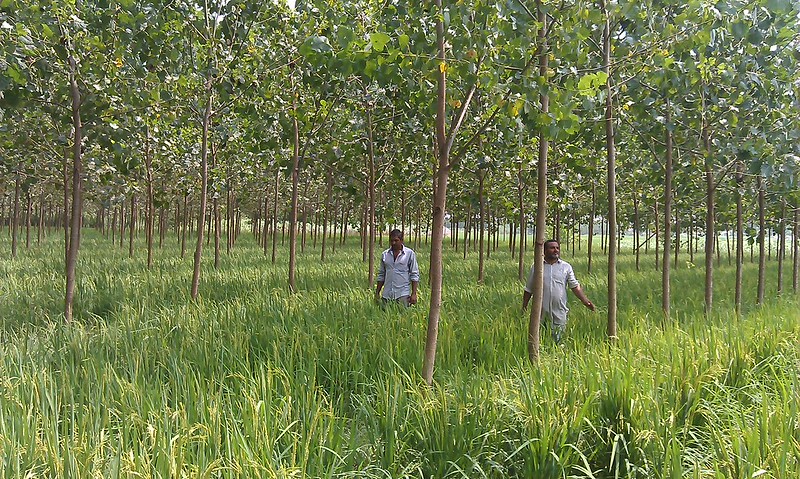
‘Resilience’ describes whether a farmer (and her farm) is able to withstand or recover from stresses and shocks. ‘Stresses’ are regular, sometimes continuous, relatively small and predictable disturbances (e.g. lack of access to inputs, a declining natural resource base, climate change and poverty) while ‘shocks’ are irregular, relatively large and unpredictable (e.g. floods, droughts, heatwaves and price volatility).
For farmers to be resilient, they must be able to bounce back from these challenges and achieve previous levels of growth – rather than suffer from reduced yields over time or even worse, a collapse in their production. Climate change already poses a risk, especially to smallholder farmers in the developing world.
Can farmers be supported to help predict these stresses and shocks? Can they be helped to prevent them, buffer themselves or fight against their negative impacts? And can they adapt in ways that make them even better off and more knowledgeable as a result?
According to the government of Ethiopia, 8.2 million people are in need of humanitarian assistance due to the current drought, coupled with successive failed seasons. El Niño weather conditions and rain failure are resulting in crop harvest loss, livestock death and declining productivity, putting over 400,000 people under emergency support needs. Despite this gloomy background, districts where World Vision has implemented Farmer Managed Natural Regeneration (FMNR) are exhibiting greater resilience. FMNR means helping naturally occurring trees to return to the landscape to help to keep the soil from washing away, to shade crops and to help the land to hold water.
Compared to the adjacent districts, agricultural production of the households that applied FMNR have largely been unaffected due to high moisture retention in their soils. Rivers and hand-dug wells have sufficient water despite reduced precipitation. Income from agricultural production has increased by more than double. Fodder for livestock, wood supply, and a stable microclimate all remain intact.
Furthermore, revenues from carbon credits that farmers have earned for planting more trees cover expenses such as school fees, medication and the purchase of improved seeds, thus safeguarding the wellbeing of families. The observed impact in Ethiopia clearly shows the potential for FMNR to serve as insurance against climate change induced shocks and stresses.
Back in the 1950s, Latin America and the Caribbean experienced one of the most devastating plant disease epidemics in history. The fungus, Panama disease, wiped out large production areas of Gros Michel, the export banana variety. This fungus still remains in the soil, and threatens the livelihood and food security of millions of smallholder farmers.
Bioversity International scientists, in collaboration with partners, have been working with 18 producers in the area of Turrialba, Costa Rica, and Tola, Nicaragua, helping them to become more resilient to Panama disease. Workshops were carried out to teach farmers how to recognise the disease, and stop it spreading. Good agricultural practices were promoted, such as using disease-free planting materials, as well as organic matter application and soil health-oriented fertilization. As a result of the interventions, farmers significantly improved their knowledge about Panama disease and management. They have also shared their experiences with neighbours through group training events, farmer field days and informal exchange.
Farmers now have a toolbox of validated practices for enhanced soil health and management of Panama disease in bananas, as a strategy for protecting their livelihoods.
In Ethiopia, an estimated 12-15 million livestock keepers live in the dry, low rangelands that cover most of the country. These rangelands have huge untapped potential, but drought, unsuitable farming practices and overgrazing have left the land in poor condition, which in turn has impacted the health, condition and value of livestock. Men, who are typically responsible for livestock production, are moving further afield in search of resources, taking them away for longer and increasing risks to their herds of disease and starvation. This affects household incomes, and results in distress sales or consumption of livestock during the hunger period, leaving many households unable to restock herds and lacking savings to invest in alternative incomes.
Farm Africa is working with partners to find more sustainable ways to use available grass and water, and to improve pasture quality. This process can be difficult to measure, as typically the areas in question are very large and remote.
The RaVeN monitoring tool under development by LTS International as part of this effort, aims to address this problem. This new tool uses freely available optical and radar satellite data in combination with meteorological data to measure the “greenness” of an area at different points in time, and therefore improve information on what good quality pasture is available for pastoralists to use for grazing their herds.

A new initiative being pioneered by scientists at the International Water Management Institute is channelling surplus surface water from flood‐prone rivers, to a modified village pond. Brick structures in the pond allow the water to flow swiftly down below ground, where they infiltrate the local aquifer. This water can then be pumped back up again during the dry season so that farmers can maintain or intensify their crop production.
Putting this into practice will save on the large funds spent each year on relief and restoration efforts of flood victims and on subsidies for groundwater extraction during the non‐rainy season.
With floods being a common occurrence across the Ganga basin, researchers hope that the scaling up of this intervention would help in effectively protecting lives and assets downstream, boosting agricultural productivity and improving resilience to climate shocks at the river basin scale. This will be especially important to help communities deal with climate change which is likely to bring ever more variability in water supply and rainfall.
Planting fruit trees is not a new practice in Central Viet Nam. Local species of pomelo and orange were once popular in home gardens and known for their special flavour. But as focus shifted towards extracting resources from the nearby forests, these fruit-bearing trees were slowly forgotten. But in the last decade, declining soil and water quantity, reduced river flow, and drought have forced farmers to seek alternatives. Tree planting in home gardens and sloping lands provides one such solution.
The World Agroforestry Centre (ICRAF), in collaboration with partners and local people has established 12 agroforestry systems in home gardens and sloping land in three villages. The systems combine trees, annual crops and fodder grass. Pomelo and orange trees are planted amid annual crops, such as beans, peanut, sweet potato, maize and guinea fodder grass.
Mixed systems are not only more resistant to climate-related hazards but recent scientific findings show that local people residing in areas with diversified agricultural or forest products are also healthier owing to more nutritionally diverse diets.
Market Access
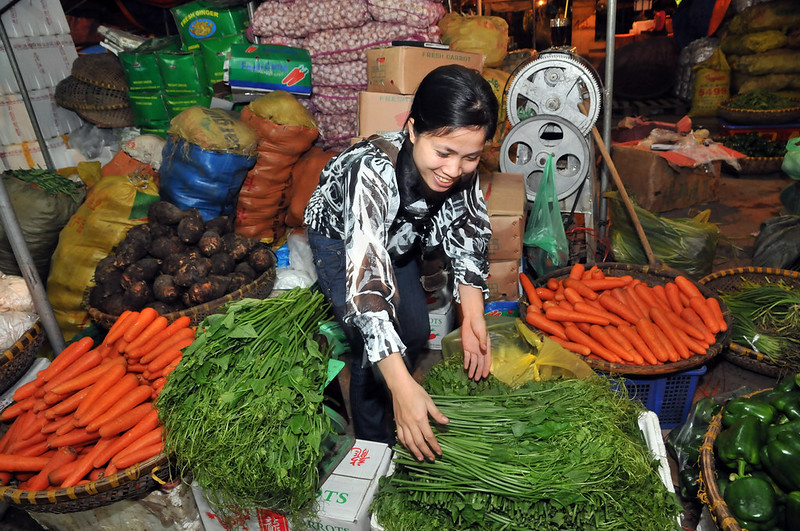
Market access allows farmers to buy the inputs they need such as improved seeds and fertilizers, and also to bring their crops, livestock and fish to market to earn a living.
Millions of smallholder famers live in remote areas, and are often isolated from market opportunities. Innovations in connecting these farmers to market are happening in many ways – resulting from social, technical and scientific advances. These advances help farmers find and share up-to-date market pricing information; protect and add value to their harvests; invest in their business; reduce and share risk; and access finance and training.
These innovations can be used and accelerated by actors all across the agricultural value chain to reduce transaction costs and risk while helping to give farmers equal access to the opportunities that exist through trade.
In Cambodia, traditional wood-burning stoves used to smoke freshwater fish typically result in low profits and emissions harmful to the environment. To improve this process and fetch higher prices from buyers, many young women engaged in this livelihood are taking part in the Cambodia HARVEST programme, funded by the United States Agency for International Development (USAID), that provides a new, fuel-efficient alternative. Eco-friendly stoves designed by the programme use 30 per cent less wood while smoking fish 15 per cent faster than conventional models. The end product is of a higher quality and ensures greater market access.
All 289 of the programme’s fish processors utilize these new stoves. Kry Sokly, a fish processor in in Kampong Prak village, has increased her family’s annual income by 75 per cent, from $1,000 to $1,750. Not only has the new stove contributed to this success, but Kry also took part in trainings on entrepreneurship and hygiene within her producer/savings group. These organizations, formed by Cambodia HARVEST as another way to connect fish processors to the market, offer an opportunity for women to come together for greater knowledge exchange. Moreover, members contribute money into a pool from which they can borrow when needed at interest rates lower than commercial lenders without stipulations on how they use the money.
In the Republic of Georgia, the agriculture sector is booming. Producers are required to adapt and utilise new technologies to keep up with both local and international market demand.

The company Herbia had run a consolidation centre, where local farmers could bring their produce to market for several years, as well as a three-hectare greenhouse for culinary production. Yet the company was in need of new technologies to increase its sales and market share, so applied to the USAID Restoring Efficiency to Agriculture Production (REAP) matching grant programme, and established a new refrigerated warehouse with two modern packing lines.
This new equipment quickly enabled Herbia to purchase more goods from smallholders and to launch a new product line that provides whole vegetables for ready-made salads. Additionally, REAP assisted Herbia in rebranding including the development of a new logo and packaging.
The new brand launched in April 2015 in more than 80 Tbilisi supermarkets, resulting in an immediate rise in sales of more than 20 per cent. The new equipment, coupled with Herbia’s rebrand, has produced 16 new jobs (including nine for women), generated more than U.S. $222,690 in sales, and enabled the purchase of more than 44MT of new herbs and vegetables from more than 150 new farmers.
Hidden in the conflict-ridden borderlands of Colombia and Ecuador, farmers have been growing exceptional quality coffee beans, but have remained largely disconnected from gourmet coffee markets. Scientists at the International Center for Tropical Agriculture (CIAT) joined forces with Catholic Relief Services last year, to analyse the coffee trade and find out how coffee farmers in the Nariño region could be linked to these more lucrative markets.
It was soon discovered that buyers from big coffee brands were purchasing Nariño’s coffee based on sight and not a taste test. Farmers were receiving a flat rate for any coffee beans considered to score above 85 out of 100, even though many, when tasted, could actually reach the high 90s. A “cupping” session was arranged by the project, to teach farmers about the rigorous tasting process that could set their coffees apart and help them earn much higher financial rewards.
In its first year, the project enabled around 100 farmers to break into the gourmet coffee market. This year they are up to around 550 and that number is likely to rise.
Traditional business model analysis dictates that the agricultural sector across Africa represents substantial risk. So it is no surprise that existing financial institutions have only met 1 per cent of the overall demand for credit in agriculture. Umati Capital focuses on data and technology, to help small to medium sized enterprises and agribusinesses unlock cash for immediate growth but also achieve operational efficiencies for sustained growth.
Umati Capital has been working closely with one of the leading fair-trade and organic certified Kenyan exporters of macadamia and cashew nuts. Before Umati Capital, the exporter painstakingly procured raw nuts from 60,000 smallholder farmers in remote areas across Kenya using manual and paper-based processes, resulting in errors and delayed payments to the farmers.
Umati Capital helped the exporter by providing invoice discounting, and automating the exporter’s supply chain processes, enabling on-time payments for the farmers.
As a result, the exporter increased purchases from farmers by 50 per cent and improved efficiencies in procurement by 90 per cent.
The MilkIT innovation platform has helped women stuggling to make ends meet in the Himalayan hills of Northern India to generate a regular income from milk from their cows.
Beginning in early 2013, the MilkIT project made efforts to unite dairy development actors, researchers and farmers, to improve access to dairy markets and improved dairy feeds, Now, more than 800 households are selling their milk at higher prices due to collective marketing by self-help group-based cooperatives and closer links to the state cooperative, with subsidies provided to those transporting milk from distant villages to markets.
Livestock keepers have been able to replace unproductive stock with higher yielding animals due to credit support provided by development. Simple feed innovations such as feed troughs, forage choppers suited to women’s needs, adoption of improved forage varieties and dual-purpose crops that act as feed and food, has helped to reduce women’s labour while increasing the availability of fodder.
An impact study conducted in November 2014 showed that families participating in this innovation platform earned five times more income from their dairy animals than non-participants in one year.
PHOTO CREDITS: ©2017 CIAT/NEIL PALMER, ©2021 CIAT/JUAN PABLO MARIN GARCÍA, YUSUF AHMAD (ICRAF), ©2016CIAT/GEORGINASMITH, DEVASHREE NAYAK (ICRAF), ©2009CIAT/NEILPALMER, GEORGINA SMITH (CIAT), S. STORR (CIMMYT), GUILHEM ALANDRY, P. SAVADOGO (ICRAF), NEIL PALMER (CIAT), OLIVIER GIRARD (CIFOR), THOMAS LUMPKIN (CIMMYT)
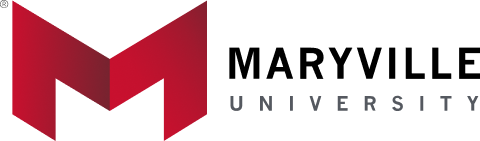
- Bachelor’s Degrees
- Master’s Degrees
- Doctorate Degrees
- Certificate Programs
- Nursing Degrees
- Cybersecurity
- Human Services
- Science & Mathematics
- Communication
- Liberal Arts
- Social Sciences
- Computer Science
- Admissions Overview
- Tuition and Financial Aid
- Incoming Freshman and Graduate Students
- Transfer Students
- Military Students
- International Students
- Early Access Program
- About Maryville
- Our Faculty
- Our Approach
- Our History
- Accreditation
- Tales of the Brave
- Student Support Overview
- Online Learning Tools
- Infographics
Home / Blog
Why Is Agriculture Important? Benefits and Its Role
July 12, 2022
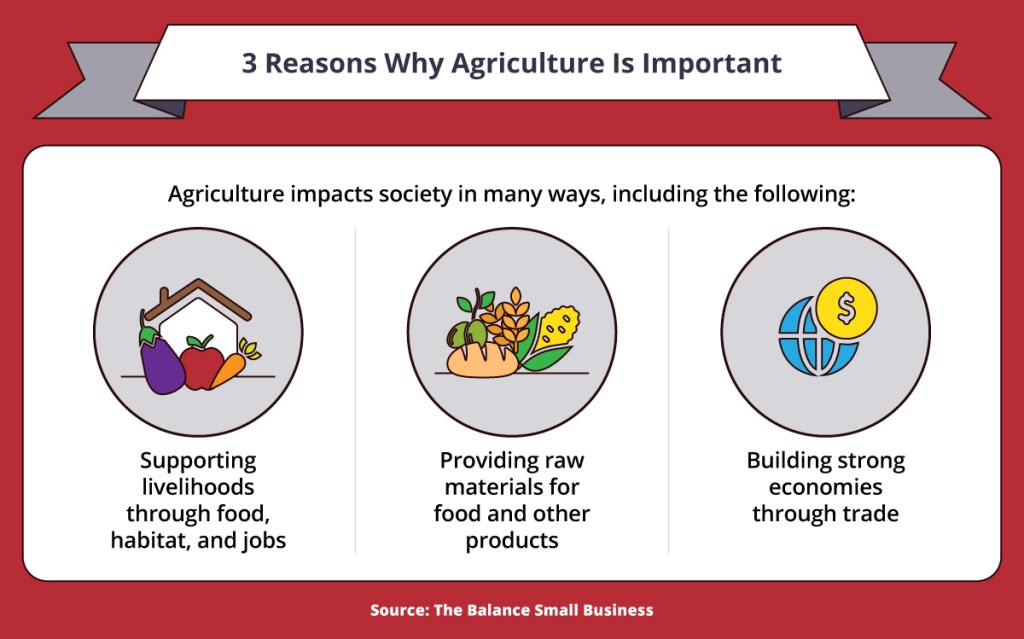
Tables of Contents
What Is Agriculture?
Why is agriculture important, how is agriculture important, importance of agriculture in everyday life, how does agriculture affect the economy, importance of agricultural biodiversity, why is agriculture important for the future.
When people think of agriculture, they often envision crop farming: soil and land preparation and sowing, fertilizing, irrigating, and harvesting different types of plants and vegetation.
However, according to the U.S. Census Bureau’s North American Industry Classification System (NAICS) , crop farming is just one element of the Agriculture, Forestry, Fishing, and Hunting sector. Agriculture also encompasses raising livestock; industrial forestry and fishing; and agricultural support services, such as agricultural equipment repair and trucking operations.
Why is agriculture important? It helps sustain life by providing the food we need to survive. It also contributes $7 trillion to the U.S. economy. Despite agriculture’s importance, the Economic Policy Institute reports that farmworkers are among the lowest-paid workers in the U.S.
However, agriculture also provides opportunities for economic equity and helps people prosper around the world. For example, since 2000, the agricultural growth rate in Sub-Saharan Africa has surpassed that of any other region in the world (approximately 4.3% annually), contributing to the region’s economic gains, according to the United States Agency for International Development (USAID). While there’s been a global decline in agricultural jobs — from 1 billion in 2000 to 883 million in 2019, according to employment indicators from the Food and Agriculture Organization of the United Nations — agriculture remains the second-highest source of employment (26.7% of total work).
Study the impact of access to natural resources, such as water, air, plants and animals
The online BS in sustainability from Maryville University will prepare you to build a better, more sustainable future. Accelerate your degree completion with our transfer credit-friendly program. No SAT or ACT scores required.
- Engage in a range of relevant course topics, from biology to business statistics.
- Learn how human health is connected to the environment through environmental science and health courses.
Agriculture is the practice of cultivating natural resources to sustain human life and provide economic gain. It combines the creativity, imagination, and skill involved in planting crops and raising animals with modern production methods and new technologies.
Agriculture is also a business that provides the global economy with commodities: basic goods used in commerce, such as grain, livestock, dairy, fiber, and raw materials for fuel. For example, fiber is a top crop in U.S. agricultural production , according to The Balance Small Business, and a necessary commodity for the clothing sector.
Back To Top
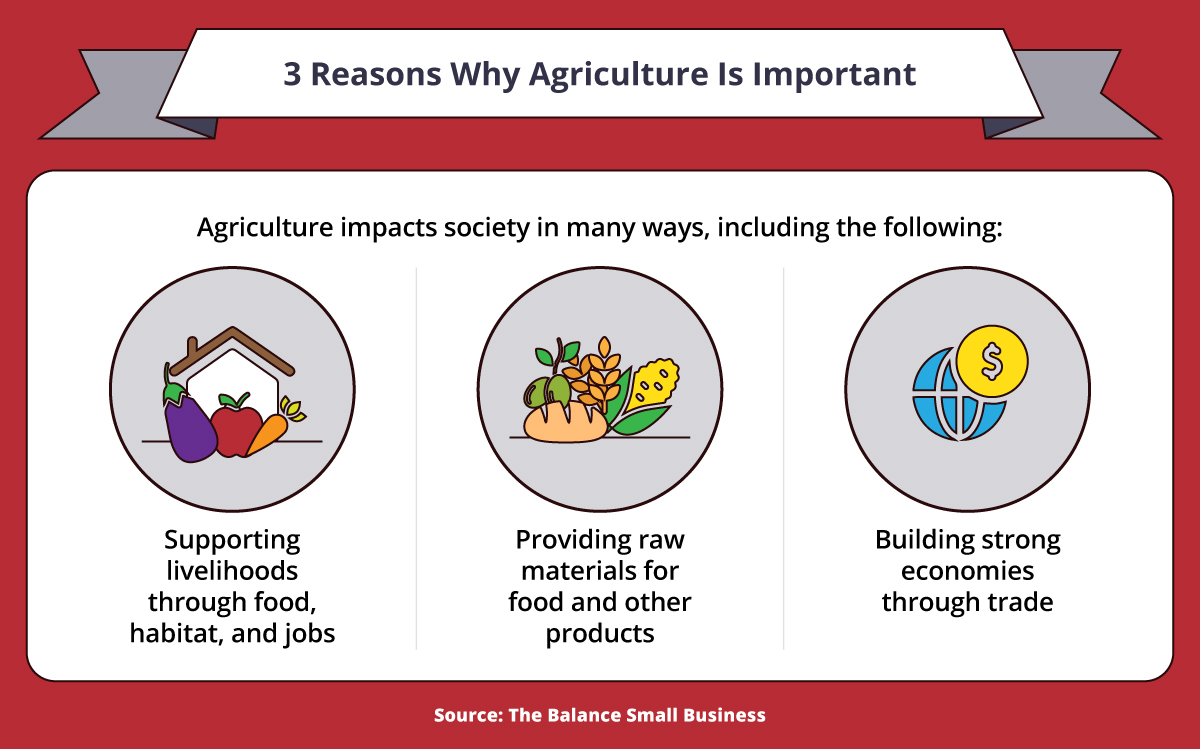
Agriculture impacts society in many ways, including: supporting livelihoods through food, habitat, and jobs; providing raw materials for food and other products; and building strong economies through trade. Source: The Balance Small Business.
A key to why agriculture is important to business and society is its output — from producing raw materials to contributing to the global supply chain and economic development.
Providing Raw Materials
Raw materials are a core building block of the global economy. Without access to raw materials, manufacturers can’t make products. Nonagricultural raw materials include steel, minerals, and coal. However, many raw materials derive from agriculture — from lumber for construction materials to herbs for adding flavor to food. Corn, for example, is used to produce foods and serves as a foundation for ethanol, a type of fuel. Another example is resins : plant products used in various industrial applications, such as adhesives, coatings, and paints used in construction.
Creating a Strong Supply Chain
Importing and exporting goods such as agricultural products requires shipping methods such as ocean freight, rail, and trucking. Delays in shipping agricultural products from a Los Angeles port can create problems in China, and vice versa, impacting the global supply chain.
For example, sales of soybean crops from Iowa skyrocketed in 2021 due to various factors including delays in South American crop shipments, according to the Iowa Soybean Association. In this example, Iowa benefited from a competitive standpoint. However, delays in shipping crops could also be detrimental to regions expecting shipment, limiting availability of products on store shelves and affecting livelihoods.
Encouraging Economic Development
Agriculture impacts global trade because it’s tied to other sectors of the economy, supporting job creation and encouraging economic development. Countries with strong agricultural sectors experience employment growth in other sectors, according to USAID. Countries with agricultural productivity growth and robust agriculture infrastructure also have higher per capita incomes, since producers in these countries innovate through technology and farm management practices to boost agricultural productivity and profitability.
Resources on the Importance of Agriculture
The following resources provide information about the importance of agriculture as a source of raw materials and its impact on transportation and contribution to economic development:
- American Farm Bureau Federation, Fast Facts About Agriculture & Food : Provides various statistics demonstrating why agriculture is important.
- The Western Producer, “Suddenly Agriculture Is Important ”: Highlights agriculture’s role as a stable commodity provider even amid disruption.
- LinkedIn, “What Is Agriculture and Its Importance? ”: Discusses the importance of agriculture in 10 areas.
When global supply chains are disrupted , considerable attention is given to the technology sector. For example, the lack of computer chips — made from silicon, a nonagricultural raw material — limits a manufacturer’s ability to make computers, cars, and other products. This impacts many areas of society and business.
Agriculture also plays a central role in meeting consumer and business market demand in a world with interconnected economies. Here are different types of products derived from agriculture.
Fruits and Vegetables
Fruits and vegetables are essential sources of fiber, proteins, and carbohydrates in human diets. Vitamins, such as A, C, and E, and minerals, such as magnesium, zinc, and phosphorus, are naturally occurring in many fruits and vegetables. In addition to health benefits, fruits and vegetables add flavors to the human palette.
Animal Feed
Some fruits and vegetables are grown to provide feed for animals, from poultry to livestock. The American Industry Feed Association reports that about 900 animal feed ingredients are approved by law in the U.S. These include ingredients that come from agricultural production, including hay, straw, oils, sprouted grains, and legumes.
Natural Rubber Production
The number of vehicles in the world is more than 1.4 billion, according to Hedges & Company market research. Every single one runs on rubber tires. According to GEP, the top rubber-producing countries are Thailand, Indonesia, and Malaysia — collectively representing approximately 70% of global natural rubber production — and about 90% of suppliers are small-scale farmers.
Cotton for Clothing
From cotton to clothes, the journey starts with agricultural production. Cotton is grown, harvested, and then processed, spun, and woven into fabric before it becomes a piece of clothing. Cotton production encompasses an expansive global supply chain, and according to Forum for the Future , it’s a leading commodity, making up approximately 31% of all textile fibers globally.
The U.S. Environmental Protection Agency (EPA) reports favorable economics of biofuels , produced from biomass sources including agricultural products such as corn, soybeans, sugarcane, and algae. The benefits include reduced greenhouse gas and pollutant emissions and the potential for increased incomes for farmers. However, biodiesel production requires the use of land and water resources that can affect food costs.
Industrial Products
Bio-based chemistry involves using raw materials derived from biomass to develop industrial products. Different industrial products derived from bio-based chemicals include bioplastics, plant oils, biolubricants, inks, dyes, detergents, and fertilizers. Bio-based chemicals and products offer an alternative to conventional products derived from petroleum products. Bio-based chemistry is considered a type of green chemistry because it promotes the reduction of environmental impacts in industrial production.
Pharmaceutical Products
For thousands of years, humans have turned to plants to help treat what ails them. For example, ginger, a plant root typically consumed in tea, can help aid digestion. Substances derived from plants and herbs can also help in healthcare. For example, extracted chemicals from the foxglove plant are used for digoxin, a drug used for heart failure. Another example is polylactic acid (PLA), a chemical produced when glucose is fermented into lactic acid in green plants. PLA has applications in tissue engineering, cardiovascular implants, orthopedic interventions, cancer therapy, and fabrication of surgical implants, according to a study published in Engineered Regeneration .
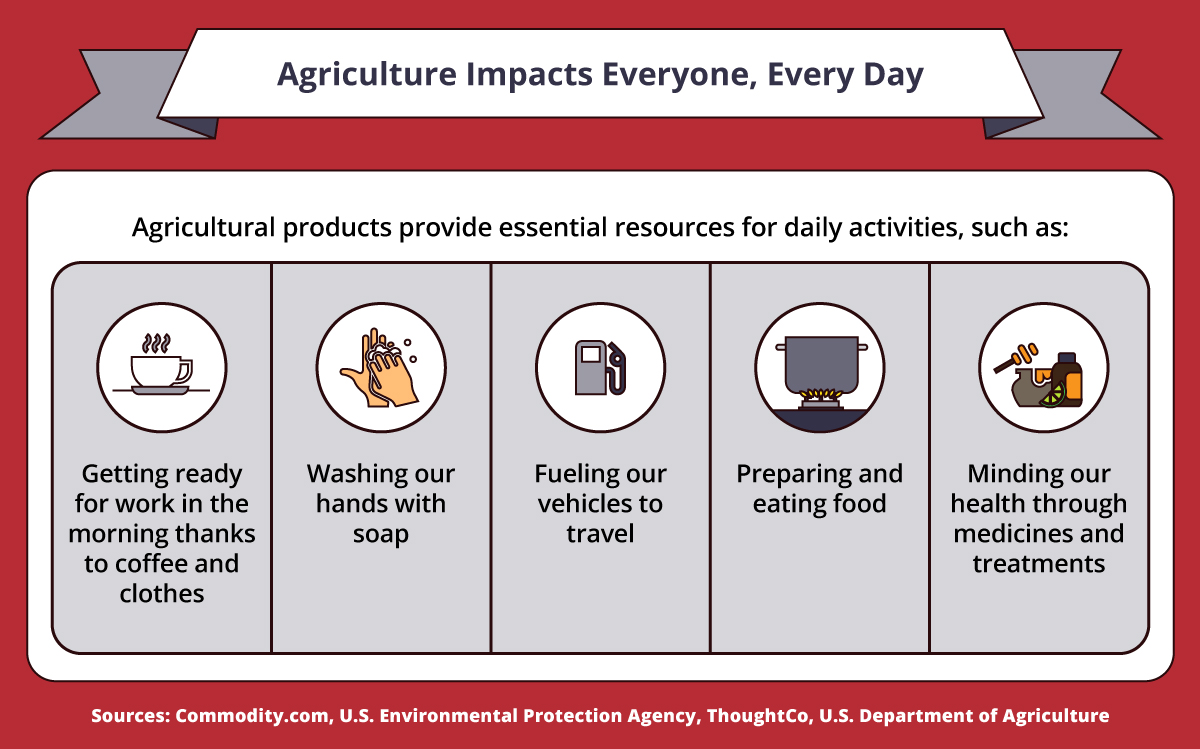
Agricultural products provide essential resources for daily activities, such as: getting ready for work in the morning, thanks to coffee and clothes; washing hands with soap; fueling vehicles to travel; preparing and eating food; and minding health through medicines and treatments. Sources: Commodity.com, the U.S. Environmental Protection Agency, ThoughtCo, and the U.S. Department of Agriculture.
For thousands of years, agriculture has played an important role in everyday life. Before agriculture, hunting and gathering enabled humans to survive. It wasn’t until the transition to the planned sowing and harvesting of crops that humans began to thrive. Humans developed tools and practices to improve agricultural output with more efficient means of sustaining themselves. From there, innovations that created industries led to the modern era.
Today, the importance of agriculture in everyday life can’t be minimized. Without the agriculture sector, activities such as getting dressed for work and cleaning the home wouldn’t be possible. Here are examples of the agricultural products we use in our everyday lives:
- Shelter . Wood and plant-based materials, such as bamboo, can be used for indoor décor and construction materials.
- Morning routine. Mint is often an ingredient in toothpaste, adding flavor while brushing your teeth, and the caffeine in coffee that keeps you awake is derived from the coffee bean.
- Dressing up. In addition to cotton, clothing can be manufactured from hemp, ramie, and flax. Bio-based materials can be used to produce grooming products such as skin creams and shampoos.
- Cleaning. Two types of chemicals used in detergents, cleaning products, and bath or hand soap — surfactants and solvents — can be produced from biomass.
- Driving to work. Plants make it possible to get to and from work. Think of rubber (sourced from rubber trees) and biodiesel fuel, which often includes ethanol (sourced from corn).
- Entertainment. Paper from trees enables you to write, and some musical instruments, such as reed instruments, require materials made from plants.
- Education. From pencils (still often made of wood) to paper textbooks, students rely on agricultural products every day.
Agriculture can have a significant effect on the economy. The U.S. Department of Agriculture (USDA) Economic Research Service reports that agricultural and food sectors provided 10% of all U.S. employment in 2020 — nearly 20 million full- and part-time jobs. Additionally, the USDA reported that cash receipts from crops totaled nearly $198 billion in 2020. Animal and animal product receipts weren’t far behind in 2020, totaling $165 billion.
The interdependence of the food and agriculture sector with other sectors, including water and wastewater systems, transportation systems, energy, and chemical, makes it a critical engine for economic activity, according to the Cybersecurity and Infrastructure Security Agency (CISA).
Agriculture also impacts economic development by contributing to the overall U.S. gross domestic product (GDP), directly and indirectly. It does so through farm production, forestry, fishing activities, textile mills and products, apparel and food and beverage sales, and service and manufacturing.
- Farm production. The latest USDA data on farming and farming income report the U.S. had a little over 2 million farms, encompassing 897 million acres, in 2020. Farm production includes producing fruits, vegetables, plants, and varieties of crops to meet demand for agricultural products throughout the country and abroad.
- Forestry and fishing activities. Agricultural activities include forestry and harvesting fish in water farms or in their natural habitat. Agroforestry is focused on “establishing, managing, using, and conserving forests, trees and associated resources in a sustainable manner to meet desired goals, needs, and values,” according to the USDA. A form of fishing activity known as aquaculture involves the production of fish and other sea animals under controlled conditions to provide food.
- Textile mills and products. The S. cotton industry produces $21 billion in products and services annually, according to the USDA. The industry has created various employment roles, such as growers, ginners, and buyers working on farms and in textile mills, cotton gins, offices, and warehouses.
- Apparel and food and beverage sales. Since agriculture is a business, selling products made from agricultural production is essential. A key aspect of the sales component in agriculture is to help growers build capacity and understand the market dynamics to meet the needs of customers, many of whom care deeply about Food services and eating and drinking places accounted for 10.5 million jobs in 2020, the largest share among all categories within the agriculture and food sectors, according to the USDA.
- Manufacturing. Agricultural products contribute to the manufacturing of a huge variety of goods, including food and beverage products, textiles, cleaning and personal products, construction materials, fuels, and more. According to the USDA, food and beverage manufacturing companies employ about 1.7 million people in the U.S.
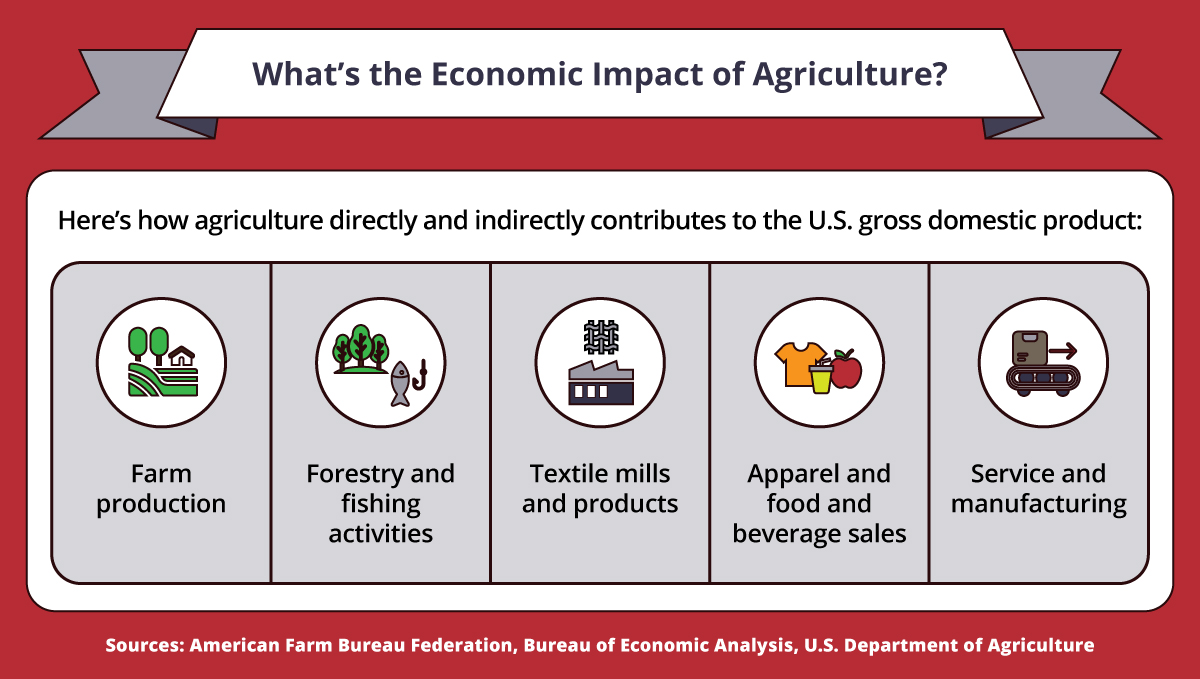
Here’s how agriculture directly and indirectly contributes to the U.S. gross domestic product: farm production, forestry and fishing activities, textile mills and products, apparel and food and beverage sales, and service and manufacturing. Sources: American Farm Bureau Federation, the Bureau of Economic Analysis, and the USDA.
Here are ways agriculture and related industries impact economic development:
Agribusiness
Agribusiness consists of the companies that perform the commercial activities involved in getting agricultural goods to market. It includes all types of businesses in the food sector, from small family farms to global agricultural conglomerates. In the U.S., farms contributed about $136 billion to GDP (about 0.6% of total GDP) in 2019, according to the USDA.
However, farms are just one component of agribusiness. Agribusiness also includes businesses involved in manufacturing agricultural equipment (such as tractors) and chemical-based products (like fertilizers) and companies involved in the production and refinement of biofuels. USDA data reports that in total, farms and related industries contributed more than $1.1 trillion to GDP, a little over 5% of the GDP, in 2019.
The economics of agribusiness also entails building production systems and supply chains that help maintain a country’s economic and social stability. Through the development of organizational and technological knowledge, agribusiness plays a vital role in protecting the environment and biodiversity near farms and using natural resources sustainably.
Food Security
Food security is central to the agricultural industry: Sustainable agriculture is a key to fulfilling the United Nations’s Sustainable Development Goals (SDGs), including SDG 2 : Zero Hunger . In addition to food security, the agricultural sector raises the incomes among the poorest communities up to four times more effectively than other sectors, according to the World Bank.
Job Creation
Throughout the world, agriculture plays an important role in job creation. For example, agriculture accounts for 25% of exports in developing countries in Latin America, about 5% of their regional GDP, according to a report about the importance of agribusiness from BBVA, a corporate and investment bank. This activity is a source of economic activity and jobs in these countries. In the U.S., agriculture and related industries provide 19.7 million full- and part-time jobs, about 10.3% of all employment.
Resources on the Economic Impact of Agriculture
The following resources highlight agriculture’s impact on the economy, from how disruption affects the business and the benefits of the sector to people’s livelihoods:
- Economic Research Service, Farming and Farm Income : Provides an overview of trends in farming and economic development statistics.
- American Journal of Agricultural Economics, “The Importance of Agriculture in the Economy: Impacts from COVID-19” : Highlights why agriculture is important based on the impact of COVID-19’s disruptions to the sector.
- Canadian Journal of Agricultural Economics, “Agriculture, Transportation, and the COVID-19 Crisis” : Discusses how transportation services that COVID-19 has disrupted can impact agricultural supply chains.
Advanced farming equipment and the increased use of fertilizers and pesticides have resulted in higher crop yields. At the same time, they’ve impacted the environment, contributing to soil and water pollution and climate change. NASA projects a 24% decline in corn crop yields by 2030, thanks to climate change. However, ensuring a healthy biodiversity can help mitigate the impact. Here are some factors to consider:
- Sustainable agriculture. Through sustainable agricultural practices , farmers and ranchers help ensure the profitability of their land while improving soil fertility, helping promote sound environmental practices, and minimizing environmental impacts through climate action .
- Climate change regulation. The agricultural sector produced about 10% of U.S. greenhouse gas emissions in 2019, according to the EPA. Regulation and policy changes can help promote sustainable practices in the sector and provide guidance on agricultural adaptation to address the challenges that climate change poses.
- Agriculture technology and innovation. From temperature- and moisture-sensing devices to GPS technologies for land surveys to robots, agriculture technology can result in higher crop yields, less chemical runoff, and lower impact on natural resources.
Agricultural Biodiversity Resources
Find information about agricultural biodiversity and its impacts in the following resources:
- Our World in Data, “Environmental Impacts of Food Production” : Discusses how sustainable agriculture offers a path to addressing food and nutrition issues.
- IBM, “The Benefits of Sustainable Agriculture and How We Get There” : Addresses how artificial intelligence (AI) and analytics technologies help farmers maximize food production and minimize their environmental impact.
- S. Environmental Protection Agency, The Sources and Solutions: Agriculture : Explains how agriculture can contribute to reducing nutrient pollution.
- FoodPrint, Biodiversity and Agriculture : Provides answers to what it will take to preserve the health of the planet to safeguard our own food supply.
- Brookings, “What Is the Future of Work in Agri-Food? ”: Discusses the future of agricultural automation and its impact on work.
Agriculture offers an opportunity to improve the lives of millions of food-insecure people and help countries develop economies that create jobs and raise incomes. Today’s agriculture also impacts future generations. To ensure the long-term success of the global agricultural sector, building a more sustainable economic system aligned with the U.N.’s Sustainable Development Goals is a crucial imperative to help create a more equitable society.
Infographic Sources
American Farm Bureau Federation, “Farm Contribution to Agricultural GDP at Record Low”
Bureau of Economic Analysis, “Gross Domestic Product (Third Estimate), Corporate Profits (Revised Estimate), and GDP by Industry, Second Quarter 2021”
Commodity.com, “Learn All About Agricultural Commodities and Market Trends”
Environmental Protection Agency, Commonly Consumed Food Commodities
The Balance Small Business, “What Is Agricultural Production?”
ThoughtCo, “List of Medicines Made From Plants”
USDA, Ag and Food Sectors and the Economy
USDA National Agricultural Library, Industrial, Energy, and Non-food Crops
Bring us your ambition and we’ll guide you along a personalized path to a quality education that’s designed to change your life.
Take Your Next Brave Step
Receive information about the benefits of our programs, the courses you'll take, and what you need to apply.
- Open access
- Published: 11 September 2024
Exploring the antifungal activities of green nanoparticles for sustainable agriculture: a research update
- Muhammad Atif Irshad 1 ,
- Azhar Hussain 1 ,
- Iqra Nasim 1 ,
- Rab Nawaz 1 , 2 ,
- Aamal A. Al-Mutairi 3 ,
- Shaheryar Azeem 1 ,
- Muhammad Rizwan 4 ,
- Sami A. Al-Hussain 3 ,
- Ali Irfan 5 &
- Magdi E. A. Zaki 3
Chemical and Biological Technologies in Agriculture volume 11 , Article number: 133 ( 2024 ) Cite this article
263 Accesses
Metrics details
Green nanotechnology has significant potential for use in agriculture particularly due to their antifungal properties, ability to control fungal diseases and reduce the reliance on chemical fungicides. Biotic stresses in agriculture have caused widespread damage worldwide, and green NPs provided eco-friendly alternatives to traditional chemical treatments, which are frequently toxic and harmful to the ecosystem. Green NPs could become an important tool in modern agricultural practices and environmental remediation if appropriate research is conducted to identify cost-effective production methods as well as safe and sustainable applications. In order to understand the potential of green NPs for sustainable agriculture and identify potential risks, research is ongoing into the effectiveness in agriculture sectors. Research update on green NPs is presented in this paper using data published on science direct over the last 15 to 20 years to clarify and understand the antifungal mechanisms of green metallic NPs, carbon and graphene nanotubes, nanocomposites as well as other type of nanomaterials. These green NPs are found to be more effective against pathogens on crops and humans than conventional fungicide approaches. They are very effective against fungi that affect cereal crops, including Fusarium oxysporum , Botrytis cinerea , and Candida species , etc. The green NPs developed using green synthesis methods are both cost-effective and environmentally friendly. Moreover, research is also required to identify the best methods for applying green NPs for crop production and sustainable agriculture. Furthermore, research should be undertaken to establish the most cost-effective methods of making and deploying green nanoparticles at a large field size study where there is fungal attack that diminishes agricultural output and affects global crop production.
Graphical Abstract
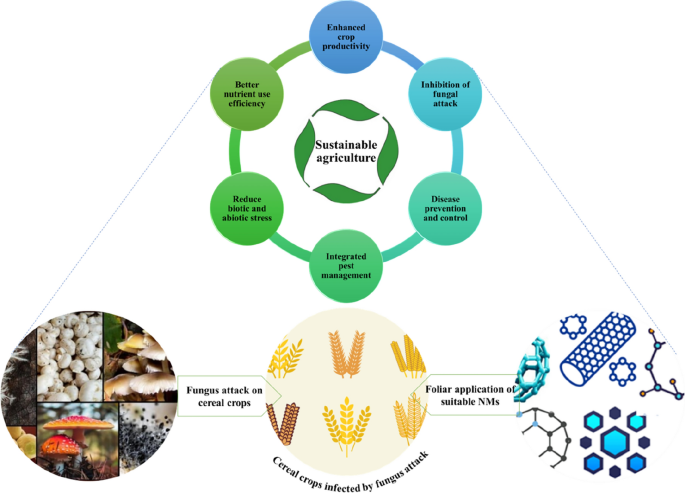
Green nanotechnology: an overview
There is an imbalance in the world's natural resources as a result of population growth and exploitation of ecosystem. Nanotechnology, according to researchers, can improve products by improving their performance, lowering manufacturing costs, and increasing resource efficiency. It is one of the most rapidly rising fields in science and technology. Nanotechnology can create nanoparticles (NPs) with higher surface-to-volume ratios and a variety of chemical and physical characteristics. Nanoparticle are widely employed in chemistry, energy, healthcare, and cosmetics for environmentally friendly applications. Metal and semiconductor NPs include oxides, nitrides, and sulphides [ 1 , 2 , 3 ]. The creation of nanoparticles by living cells, especially via plant resources is the subject of the newly developing scientific field known as “green nanotechnology”. Numerous sectors rely on this field, including electronics, biotechnology, nuclear energy, fuel and energy, and pharmaceuticals as well as for the remediation of various environmental ailments [ 4 , 5 , 6 ]. Since biological procedures using green synthesis tools are safer, more environmentally friendly, non-toxic, and more economical than other similar approaches, they are better suited for synthesizing nanoparticles between 1 and 100 nm. The top-down and bottom-up approaches use different physical, chemical, and biological processes to create the metal nanoparticles [ 7 , 8 ]. Following Fig. 1 explores the green synthesis routes along with the potential applications of green nanotechnology.
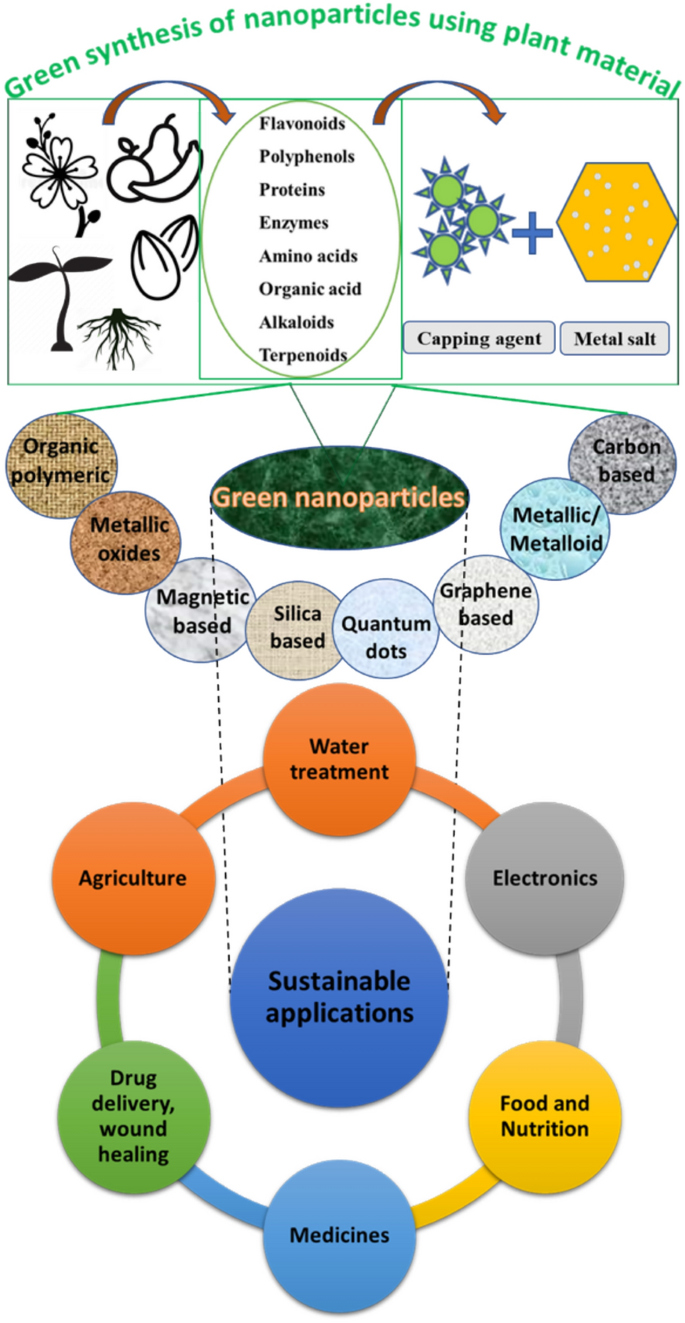
Mechanism of green synthesis of nanoparticles by using plant materials and its sustainable applications in various sectors
Greenly produced NPs have been shown to enhance the performance of solar cells, photocopiers, xerography, rectifiers, antioxidants, and photocatalysis [ 9 ]. According to Pansambal et al. [ 10 ], green-produced cerium oxide nanoparticles have antioxidant, antidiabetic, anticancer, antibacterial, and antifungal properties in addition to photocatalytic dye degradation. Potential photocatalytic, antioxidant, and antibacterial properties of green-produced stannic oxide nanoparticles make them useful for improving environmental and human health applications [ 11 ]. Applications in biomedicine and the environment are being developed with green-produced silver chloride nanoparticles [ 12 ]. Different plant parts are used to create green synthetic metal nanoparticles, which are also generated using economical, non-toxic, and environmentally beneficial processes. In contrast to different physical and chemical methods, environmentally friendly produced nanoparticles perform more actively in the removal of dyes, antibiotics, and metal ions from the soil and water media. The most effective way to make nanoparticles is by green synthesis, which also happens to be a cost-effective, environmentally friendly, and very stable process. In environmental and biological applications, green synthesis techniques respond more favorably [ 13 , 14 ]. Numerous phytochemical substances with oxidation–reduction properties, such as flavonoids, phenolics, terpenoids, and polysaccharides, are found in plants. For this reason, they are best used in the environmentally friendly creation of nanoparticles. The process of creating stable nanoparticles requires precise understanding of the phytochemical components, hence the synthesis of phytochemical compounds for nanoparticles is not a general process [ 15 , 16 ]. Most people feel that the important actors in the creation of environmentally friendly nanoparticle manufacturing are plant secondary metabolites, notably polyphenols, phenols, and other plant materials that participate in the synthesis process. According to [ 17 ], green synthesis approach is more sophisticated, repeatable, safe, and inexpensive. Comparing plant-based green manufacturing of nanoparticles to other comparable biological processes involving actinomycetes, bacteria, fungi, and algae reveals certain advantages [ 18 ]. The presence of considerable phytochemicals in these artificially manufactured green nanoparticles raises concerns for numerous plant parts, including the roots, stem, leaf, seed, and fruit [ 19 ]. In various plant portions, squeeze, wait, and apply salt solutions after cleaning with tap or distilled water to produce plant-synthesized nanoparticles. Using this method, metallic salts were added, and then the nanoparticles were eliminated using the required laboratory procedures. Among the industries that employ green nanoparticles are agriculture goods, food, aquaculture sciences, personal hygiene, medicine, and nano-enabled technology.
Green nanotechnology and agriculture
Green nanotechnology research has demonstrated a considerable potential to alleviate major barriers to reaching sustainable agricultural production objectives. Utilizing environmentally friendly materials has the potential to transform food systems and address the global food security issue of today. With the magic of nanotechnology, it has the power to change modern agriculture from the period of genetically modified crops to the exciting new era of atomically changed organisms [ 6 , 20 , 21 , 22 , 23 ]. The excessive use of chemical fertilizers for higher yields give rise to growth of insects and microbes causing fungal diseases in great numbers in the present day unsustainable agricultural practices. These fungal attacks can possibly impact both the crops growth and the crops yield imposing economic losses to the farmer’s community.
On the other hand, the innovative GNT is based upon the applications of nanotechnology principles and techniques applied in an eco-friendly mode for effective control of fungal activities of various pathogens in agriculture. The GNT involves the use of suitable materials on a microscopic scale of (0.1–100) nm size to effectively control the desired ailments from the start to the maturity of the plants. Hence, their applications in agriculture can enhance crop production and improve resource efficiency, offer innovative and eco-friendly approaches to control all possible antifungal activities in plants [ 24 , 25 , 26 ]. It is noticed during the research, that the use of GNT applications can greatly increase the stability of crops by reducing the losses due to abiotic and biotic stresses, producing higher crop yields by curtailing the production costs in agriculture [ 27 ]. The GNT involve the applications of following novel nanoparticles techniques in the field of emerging agriculture. The nano-coatings on seeds can speed up germination rates, protect against pests and diseases, and can provide controlled release of nutrients during early growth stages of plants in agriculture [ 28 ].
The nanotechnology can contribute to the development of efficient nano-based water filtration and adsorbent systems that ensure pathogen and toxic free clean water for irrigation purposes. A lot of inorganic materials, such as heavy metals, were present in the wastewater from the industries, posing serious health hazards to people. Nanobased filtration alleviates those effects. This technology can also be used to purify water from other sources, such as rivers and lakes. It can provide a cost-effective and efficient way to clean contaminated water and make it safe for consumption [ 29 , 30 , 31 , 32 , 33 , 34 ].
Numerous studies have demonstrated the potential of green nanotechnology to regulate stress-induced changes in plants. Moreover, the regulated and targeted release of nano-pesticides has been shown to be a highly successful method of removing biotic stressors in agriculture, particularly for wheat crops [ 35 , 36 ]. Some possible green NPs measures for the agriculture sector's sustainable farming practices are shown in Fig. 2 below. These actions include preserving water, enhancing soil health, and using fewer toxic pesticides and fertilizers. Using sustainable energy sources, including wind and solar energy, can also aid in lowering carbon emissions. These properties and futuristic approach of green nanotechnology can enhance the crop production in agriculture [ 37 , 38 ]. The development of nano-scale formulations for pesticides can improve their efficacy and reduce the amount of chemicals needed. Nano-encapsulation of active ingredients enhances targeted delivery and reduces the environmental contamination to the minimum level. The nano-based fertilizers aim to enhance nutrient uptake by plants, increasing the efficiency of nutrient utilization. Moreover, the controlled release mechanisms in nano-based fertilizers can supply necessary nutrients to plants for wider periods and hence reducing the need of their frequent supply to the plants. Moreover, the use of nano-carriers can improve the efficiency of delivering various agricultural inputs primarily pesticides, nutrients to the plants [ 39 , 40 , 41 , 42 ].
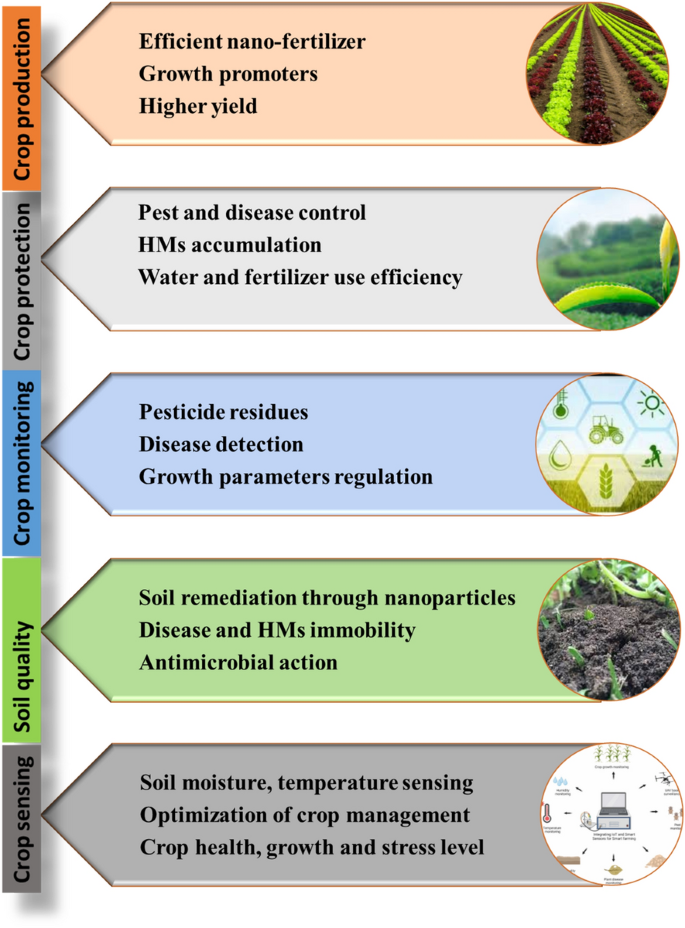
Role of green nanoparticles for the agricultural services as crop production, crop protection, crop monitoring, soil quality, and crop sensing
Antifungal activities of green nanoparticles
Techniques involving plants with nanotechnology called “green nanotechnology” offers an efficient, eco-friendly management and control of these fungal pathogens in agriculture. Plant-based nanotechnology is a cutting-edge approach that will undoubtedly bring an era of agricultural technology innovation to solve such issues. Nanoparticles based on phyto-extracts demonstrate the potential of antimicrobial activities for effective fungal pathogen control compared to conventional fungicides. In addition to ensuring plant health, nanoparticles satisfy agriculture's growing need for high output. The limits of chemicals and the potential of green nanoparticles, which provide fresh approaches to managing fungicides that cause fungal illnesses in agriculture, are the primary topics of this paper. Recent research showed that the rise of fungal diseases in plants resulted in economic losses in the agriculture. Chemical fungicide sprays are not an environmentally acceptable way to treat fungal illnesses since they pollute the environment and pose a risk to human health as well as other biotic life forms. However, these chemical fungicides appear overused due to their affordability and ease of application [ 43 ]. According to Moore et al. [ 44 ], fungi account for 70–80% of the losses brought on by microbial diseases. It is believed that there are around 1.5 million species of kingdom fungus, and most of these fungal pathogens cause plant illnesses and production losses. Fungal infections have been responsible for agricultural losses exceeding 200 billion euros annually [ 45 ]. Animal pests account for around 18% of agricultural crop losses, with microbiological diseases and weeds accounting for 16% and 34% of losses, respectively. However, it is utmost essential to consider potential environmental and health impacts while employing green nanotechnology in agriculture. The overall sustainability of agricultural practices requires careful development of GNT and essentially should be done thorough valid risk assessments before its field application.
A variety of fungal infections were effectively inhibited by the ZnO NPs made from Parthenium hysterophorus plant extracts. For example, ZnO NPs based on parthenium start to significantly slow down the growth of A. flavus and Aspergillus niger pathogens, respectively [ 46 ]. ZnO nanoparticles, which were produced with the help of Syzygium aromaticum bud extracts, shown efficacy against Fusarium graminearum , a pathogen that typically inhibits the growth of mycelial cells and the synthesis of mycotoxins such as zearalenone and deoxynivalenol. At the same time GNT treatments can raise lipid peroxidation, reactive oxygen species (ROS) production and lower ergosterol matters of fungal membrane which is highly damaging to established pathogens in agriculture [ 47 ].
Downy mildew, produced by Plasmopara viticola , reduces the crop production that effects the food security. This illness can be efficiently managed with a nano-composite of graphene oxide (GO) and iron oxide (Fe 3 O 4 ) known as GO-Fe 3 O 4 . Pretreating leaf discs with this nano-composite before inoculating with P. viticola sporangium significantly reduces spore germination, most likely by restricting water routes in the sporangia. While ordinary Fe3O4 and GO have limited control on spore germination, the nano-composite is far more powerful. Graphene oxide coated with silver nano-composite can cause antifungal effects by interacting with fungal cell membranes and disturbing their bonding severely as in case of pathogen F. graminearum [ 48 ].
The use of silver nanoparticles (Ag NPs) has demonstrated a favorable impact by establishing direct contact between the Ag ions and the pathogen’s spores and germ tubes, so stopping their negative influence. This confirms that Ag NPs are capable of curbing a variety of illnesses caused by plant pathogenic fungi. So AgNPs provide strong antifungal properties by disrupting fungal cell membranes and halting their cellular processes for further growth. They are effective against a broad spectrum of fungi, making them valuable in agriculture [ 49 ]. The Ag NPs are commonly utilized for sterilization processes, such as waste-water treatments and water sanitization, because of their antimicrobial qualities [ 50 ]. By employing the green chemistry approach, Ag NPs may be synthesized to regulate the detrimental effects of certain fungal diseases. For example, Krishnaraj et al. [ 49 ] tested the efficacy of Ag NPs at varying concentrations against a variety of fungal plant pathogens, such as Rhizoctonia solani , Macrophomina phaseolina , Alternaria alternata , Curvularia lunata , Botrytis Cinerea, and Sclerotinia Sclerotiorum , using green AgO NPs utilizing the leaf extract of Acalypha indica . Amazingly Ag NPs with a concentration of 15 mg showed a remarkable inhibitory activity against all above pathogens in the field of agriculture. In a different study, Ag NPs (30 ppm) prepared from AgNO 3 (5 mM) solution using Argemone mexicana leaf extract were shown to be extremely poisonous to the pathogenic fungus Aspergillus flavus [ 51 ]. Also, Ag NPs can be manufactured by using seeds extract of T. peruviana (10%) mixed in chemicals of AgNO 3 (1 mM) in the presence of sunlight or autoclave method or combination of both techniques. The performance of Ag NPs is much bigger by careful treatment which may inspire the direct contact of Ag ions with germ tubes and spores to control effectively pathogen and fungi activities in agriculture [ 49 ]. The following Table 1 illustrates the use of several NPs for successfully reducing fungal attacks on different crops. The NPs used are commercially available and have proven their effectiveness in reducing the attack of fungi on crops. These NPs are applied directly to the plants, where they act by inhibiting the growth of fungi. In some cases, the NPs can also be used to prevent future fungal attacks.
In the field of plant pathology in agriculture, the green NPs may be effectively utilized to treat a range of fungal infections [ 106 , 107 ]. Kumar et al. [ 108 ] reported on the use of Aloe Vera ( Aloe barbadensis Miller ) leaf extract for the production of Cu NPs, which shown antioxidant properties for plant diseases, including blackberry fruit. Also use of Citron juice (The Citrus Medica) for the biosynthesis of Cu NPs confirmed strong inhibitory properties against the pathogens of F. graminearum , Fusarium , culmorum , F. oxysporum and culmorum Fusarium , respectively. However, they proved less effective against pathogens of F. graminearum and F. oxysporum , respectively [ 109 ].
The study of green synthesis of Cu NPs with the stem extract of clove ( Syzygium aromaticum ) displays an outstanding antifungal action against pathogens Aspergillus niger , Aspergillus flavus and Penicillium spp., respectively [ 110 ]. Further successful control of fungal activities by green Cu NPs was reported against the harmful phytopathogens including Penicillium digitatum, Fusarium oxysporum, Phoma destructiva, Phytophthora cinnamon, Alternaria alternata, Pseudomonas, Curvularia lunata, syringae, and Alternaria alternata , respectively [ 111 ].
The gold nano particles (GNPs) can be successively synthesized by green method using variety of fresh leaves extract of Memecylon edule [ 112 ], Punica granatum [ 74 ], Capsicum annuum [ 113 ], Magnolia kobus and Artemisia dracunculus [ 114 ]. They are also synthesized by floral excerpts of Moringa oleifera [ 115 ]. These green nanoparticles have also an effective antifungal agent when mixed with GNPs [ 116 ]. GNPs were found more effective when used with suitable non-toxic reducing agents, especially sodium boro-hydride and sodium citrate, respectively [ 117 ]. According to Mittal et al. a range of stabilizing and reducing agents for the management of fungal infections in agriculture may be made from plants [ 118 ]. Therefore, to prevent fungal illnesses, non-toxic, healthful, and environmentally friendly sources must be developed [ 119 , 120 ]. Fungicides made of synthetic chemicals are poisonous and harmful to the environment, soil biodiversity, and human health. Accordingly, trends are changing in favor of using NPs to safely and effectively treat fungal infections in plants. It has been discovered that organic and inorganic NPs with a variety of biological purposes are effective against bacterial, viral, and fungal infections. Plant extracts are the most significant biological material bio-reductant for the creation of NPs [ 121 ]. Since phyto-extracts control fungal infections, encourage plant development, and successfully lower agricultural illnesses, they may be utilized to synthesize environmentally friendly NPs [ 122 ]. It is discovered that the several “green” produced NPs are lucrative, non-toxic, easy to use, and inexpensive. They are appropriate for curing agricultural plants of diseases. Compared to the several old approaches, the green synthesis using NPs produced more stable synthesized materials and is an essential component of agricultural sustainability [ 123 ].
Green nanoparticle manufacturing and use are likely to increase due to rising environmental consciousness, regulatory pressure to eliminate hazardous waste, and demand for sustainable solutions. The three categories of green nanoparticle synthesis are phytochemicals, extracellular, and intracellular. Due to the availability of phytochemical components in the extract, which can also function as reducing and stabilizing agents to turn metal ions into metal nanoparticles, the process of producing metal nanoparticles from plant extract is low cost and high yield [ 124 ]. Green nanoparticles, a fast-expanding sector, are experiencing substantial development due to increased demand for sustainable solutions across a variety of sectors. Global green nanotechnology market, estimated to increase from 2020, is expected to grow more by 2030, with high contributions from various countries of the world. The consumption and production of green NPs synthesized by eco-friendly methods are growing rapidly, as industries seek sustainable alternatives for their businesses. Green nanotechnology global market, estimated valued at $8.3 billion in 2020, will be projected to reach $26 billion till 2028, with significant contributions from the Asia-Pacific region. The main contributions of green NPs are mainly used in environmental remediation, agriculture, medicine, and with the healthcare sector driving substantial growth [ 125 , 126 , 127 ]. Environmental and agricultural applications are also expanding and reflect huge demands for sustainable solutions across these industries.
The global commercial production of green NPs faces challenges in scaling up despite growing interest in sustainable synthesis methods. Plant-based green synthesis has been proposed as an alternative, it has yet to achieve large-scale commercial viability [ 128 ]. Green nanoparticles are rapidly being employed in health, agriculture, and environmental remediation, with considerable market growth predicted in these sectors. Nanoparticles are found in both organic and inorganic modules, including ferritins, liposomes, micelles, dendrimers, and magnetic NPs, as well as metal and semiconductor NPs such as oxides, nitrides, and sulfides [ 129 ]. These green nanoparticles are sprayed as foliar treatments to the targeted crops to reduce disease. Overall, nanoparticle-based treatments are potential alternatives to traditional fungicides for controlling plant diseases in a variety of crops [ 130 ].
Antifungal activity of other nanocomposites synthesized by conventional methods
The overuse of pesticides and other chemicals, along with conventional methods for nanoparticle synthesis, has detrimental impacts on soil fertility, soil microorganisms, and the health of people, plants, and animals. By altering metabolic and physiological processes, the increasing use of conventional fertilizers has led to the emergence of pathogen strains that are resistant to them and delays the growth of photosynthetic pigments and plant reproductive organs. They also prevent plants from going through mitosis, forming microtubules, and respiring their cells. Engineered nanoparticles exhibit promise antifungal effectiveness against a variety of fungal species, including drug-resistant Candida albicans. Silver nanoparticles (Ag-NPs) have considerable antifungal activity, equivalent to traditional antifungal treatments [ 131 ]. Polyvinylpyrrolidone-coated Ag-NPs, when coupled with azole antifungals, have synergistic effects on resistant C. albicans , compromising cell membrane integrity and preventing budding processes. Amphotericin B-conjugated silica nanoparticles have fungicidal action against Candida sp. and may be reused repeatedly without losing efficacy [ 132 ]. Sub-lethal doses of different nanoparticles, such as Ag, SiO 2 , TiO 2 , and ZnO, might improve the antifungal activity of beneficial bacteria such as Pseudomonas protegens CHA0 by increasing the formation of antifungal chemicals [ 133 ]. These findings indicate that tailored nanoparticles may have significant benefits in fighting fungal infections and developing novel antifungal strategies. The research with carbon-nano tubes (CNTs) verified that multi-walled-carbon nanotubes (MWCNTs) can greatly enhances both the ability of seed germination and plant growth by control of antifungal activities. Furthermore, Tripathi and Sarkar [ 41 ] found that applying water-soluble CNTs helped wheat plants expand their roots and shoots in both light and dark environments. Additionally, it has been confirmed that industrial-grade MWCNTs (2560 mg kg −1 ) significantly increased crop germination and root elongation [ 42 ]. The following Table 2 explores the antifungal/antimicrobial action of various other nanomaterials that are being applied for antifungal activities on different crops.
Mechanism involved in the antifungal activity of green nanoparticles
Applying nanoparticles as a foliar spray on the cereal crops provide a multifaceted approach to fighting fungal infections, leveraging both direct antifungal properties and indirect benefits through soil and plant health improvement. When cells were exposed to NPs, they produced more ROS and OH radicals, reducing regulation of antioxidant machinery and oxidative enzymes, disrupting cellular integrity and osmotic balance, and decreasing pathogenicity. As a result, lipid peroxidation increased, inflammation developed, mitochondrial function declined, and cell death succeeded [ 141 , 142 ]. There was evidence that NPs caused cell death by a caspase-dependent pathway, suggesting they could induce apoptosis. As a result of NPs, ROS were generated more and antioxidant enzyme activity decreased. Antifungal effects of metallic nanoparticles are attributed to their electropositive surfaces, which oxidize plasma membranes and allow entry into the pathogen body [ 142 , 143 , 144 ]. The results of Zhang [ 145 ] provide more evidence for this, since they address the reversible conversion of Ce (III)/Ce (IV) between two valence states as a unique antibacterial mechanism. The role of ROS in the antibacterial activity of CeO 2 NPs is also highlighted by Kuang et al. [ 146 ] who found that exposure to these particles can increase intracellular ROS levels in E. coli . However, the specific mechanism of the antifungal activity of CeO 2 NPs and biochar is not fully elucidated and requires further research. It is possible that ROS generated by CeO 2 NPs are involved in the disruption of cell walls, leading to the death of fungal cells. It is also likely that ROS can activate the immune system, aiding in the fight against fungal infections. ROS may also damage the fungal membrane, preventing the transport of essential molecules such as oxygen and nutrients. Additionally, ROS can react with fungal enzymes, damaging their ability to catalyze important reactions. ROS can also damage the DNA of fungal cells, leading to mutations that prevent the cells from reproducing and spreading. Furthermore, in one of its foliar applications to wheat seedlings, ZnO NPs of nAl 2 O3 (< 50 nm) shown reducing the root length of the plants owing to oxidative stress activity of superoxide dismutase with catalase enzymes raising. The smaller concentration of ZnO NPs causes healthy impact on seed germination process. On the contrary, higher concentration of ZnO NPs can cause seed germination degradation as it is insoluble in water. The ZnO NPs display antifungal effects by inducing oxidative stress and damaging fungal cell membranes. Additionally, ZnO NPs created using phyto-extract of Eucalyptus beads were investigated to predict the fungal pathogen that causes illness in apple plants. Amazingly at 100 ppm concentrations, the highest reserve of 76.3% was noticed for pathogen Alternaria mali , 65.4% for Botryosphaeria dothidea and 55.2% for Diplodia seriata , respectively. Thus, it is possible to use these NPs to effectively control the aforementioned fungal infections in order to safeguard different fruit harvests in agriculture on time [ 74 ]. The silver-based chitosan Ag-Chit NPs possess antifungal properties due to their ability to bind to fungal cell walls, disrupting their body structure. They are found very fruitful, especially in its role as bio-fungicides in the field of agriculture. The Ag-Chit NPs were proved very effective in controlling the fungicides and pest communities of A. flavus present in the feed of livestock. These pest-suffered feed samples were collected and accordingly treated by Ag-Chit NPs composites of 30, 60 and 90 mg, respectively, for 10 days incubation at 10 °C producing successful results. Animal pests can cause agricultural harvests to drop by up to 18%, while microbiological illnesses and weeds caused losses of 16% and 34%, respectively. Fungal infections have been responsible for agricultural losses exceeding 200 billion euros annually [ 147 ]. Figure 3 provides the insightful mechanism against the fungus pathogen of wheat crop under the combined application of nano-biochar. Nanoparticles significantly increased the permeability of cells when exposed to them, resulting in alterations to their membranes.
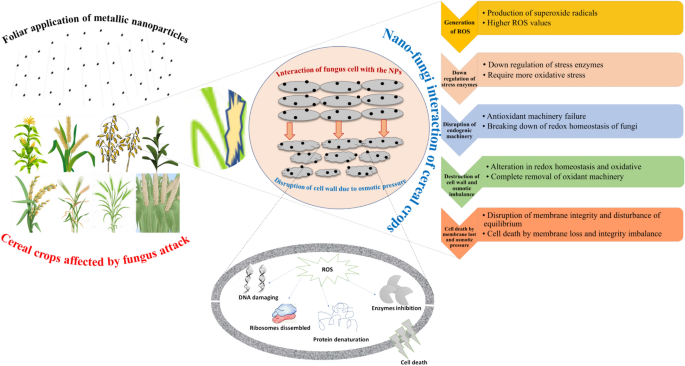
Mechanism of antifungal activities of green nanoparticles
Toxicological effects of green nanoparticles
Green nanoparticles’ hazardous behavior toward the environment and its constituent parts has not been well examined. Nonetheless, a lot of research has been done on the harmful effects of the physicochemical characteristics of artificial nanoparticles. It has been discovered that the oxidation potential, DNA damaging potential, and pharmacological behavior of smaller particles are directly correlated. Almost all cell types are harmful to particles smaller than 50 nm [ 148 ]. According to Tran et al. [ 149 ], these green nanoparticles have the ability to stay suspended in water and the air for extended periods of time, exposing living things for longer and increasing their toxicity. According to reports, endocytosis and phagocytosis are influenced by the nanoparticle's form (triangular, star, tubular, or circular) [ 150 ]. It was also discovered that endocytosing circularly shaped nanoparticles was simple and they could be endocytosed quickly and efficiently even in the presence of other nanoparticles [ 151 ]. According to Gatoo et al. [ 150 ], a particle’s surface charge has a significant influence on its agglomeration behavior and, consequently, its toxicity may increase. Furthermore, surface coatings of organic compounds can affect the pore structure, surface charge, surface roughness, reactivity, and surface roughness of green nanoparticles, depending on the type of coating. The performance of green nanoparticles in a range of applications, such as biomedical, energy, and materials, can be strongly impacted by these features. Therefore, before green nanoparticles are applied, designed, or developed, their toxicological effects should be taken into account.
Emerging trends and technologies in agriculture sector
Emerging trends and technologies in agricultural arena make use of state-of-art data-driven decision-making through latest sensors and drones for fastidious farming based on green nanotechnology. In the future, farms will be factories to meet consumer needs. With the rise of capital-intensive industries and services, artificial intelligence (AI), robotics, and machine learning are replacing humans, saving them labor. The largest obstacle facing emerging nations is the lack of well-paying jobs in the agricultural sector, including secondary agriculture, processing, packaging, value chains, and value addition. Bio-based goods are finding increasing applications in the fields of alternative energy, building materials, chemicals, polymers, pharmaceuticals, cosmetics, fertilizers, nutrition, and insect/pest control. Biofuels are made from grains, oilseeds, and sugarcane [ 152 ]. Promotion of novel CRISPR–Cas9 and other gene editing tools can aid in the development of genetically engineered crops with increased resistance and nutrient value. The development of controlled-environment agriculture through the use of vertical farming (VF) is one of the finest strategies for ensuring year-round output with lower resource consumption, particularly in urban agriculture. The widespread adoption of GNT may improve global sustainable development, and the inclusion of a block-chain system for transparent supply chains can secure the agricultural requirements of both current and future generations [ 153 , 154 ]. At the same time, the GNT innovations mutually boost the energy efficiency, food sustainability by maintaining a fair balance between environmental resources, economy and social needs of the people as per the United Nations Sustainable Development Goals (SDGs). Despite having few commercially available products, green nanotechnology is still primarily in the research and development stage, despite its potential for sustainability and environmental benefits. Even though it claims to cut hazardous waste, greenhouse gas emissions, and energy consumption, its current influence on environmental protection is negligible. However, due to their special qualities, nanomaterials are useful for lowering environmental risks, improving energy efficiency, and producing long-lasting, environmentally friendly products. These technologies are anticipated to contribute significantly to energy challenges and climate change mitigation as they develop.
In order to address the present challenges of global warming, overconsumption of natural resources, and an ever-rising population, there is need of shifting from unsustainable traditional agricultural practices, causing the growth of chemical fungicides to eco-friendlier practices for ensuring global food security. The promotion of green nanotechnology is a suitable option for sustainable management of various plant pathogens without affecting the environment. This critical review profoundly calls for the large-scale production and use of green nanoparticles synthesized by plant extracts, which can greatly enhance the quality and yields of food by curtailing the harmful effects of chemicals and fungicides in the field of present-day agriculture. Green nanotechnology should be encouraged at large-scale due to its cost-effectiveness and environment friendly properties and its ability to ensure sustainable global food supplies to achieve some of the United Nations Sustainable Development Goals.
Futuristic scope and prospective of green nanotechnology for antifungal activity
Certain concern needs to be addressed before the large-scale application of nanotechnology practices in agriculture sector. For instance, the antifungal management requires nano-hybrid materials which are merged by various combinations of gold, silver, zinc, grapheme, copper, iron, polymers say chitosan and variety of organic molecules and chemicals which are highly expensive. At the same time the nano-hybrid construction techniques require expensive high-tech devices of higher energy inputs needs can raise the production cost of nano-hybrid materials. The use of nanohybrids is mostly very effective against phytopathogens control but in actual field conditions, they sometime display off-target movements and may damage the plants by entering into the vegetative parts of the plants.
Hence, the potential impacts of various nanoparticles for antimicrobial and fungicidal applications must be fully registered before evolving the particular nano-formulations and nanohybrids for agriculture purposes. It is also noticed that most metallic/metal oxide NPs may exercise negative impacts in plants and can also alter or reduce the soil microbial levels. Marketing of GNT products is another sky-high issue for their field use on broader levels possibly due to multifarious reasons including unclear technical benefits, high cost, lack of formers/public awareness and uncertainties in legislation about GNTs. Due to these reasons the applications of GNTs in agriculture sector is minimal as compared to other sectors of social and natural sciences. The sensors/kits built of nanomaterials should be used throughout the post-harvest phase in order to promptly identify the fungal infection. The concentration of nanocomposites used in a field determines how harmful they are. When compared to the concentrations of chemical-based insecticides and fungicides, the working concentrations of nanocomposites are relatively low. The capacity of biodegradable polymers to easily translocate inside plant tissues and to have antifungal properties in plants is the ultimate goal when creating nano-composite materials using different combinations of metal and metal oxide nanoparticles. Therefore, further research on biodegradable polymers should be encouraged due to their eco-friendly and biocompatible nature, which ensures sustained formation. The time, money, and resources required to produce GNT for agriculture can be recovered by establishing biological synthesis techniques. Additionally, it may successfully reduce the quantities of environmentally hazardous chemicals needed for the commercial synthesis of non-composite materials and nanomaterials using the most well-researched physical/chemical synthesis methods accessible worldwide.
Availability of data and materials
All the data of this study is contained in the manuscript. For any additional data or information needed regarding this review article, please contact the corresponding authors.
Ghazy H, Shareef AM, Hasan AA, Alhommada MR, Alanssari AI, Jasim QN, Hawas JN. Integrating nanotechnology into digital supply chain to increase productivity and reduce environmental pollution. Nanotechnol Percept. 2024. https://doi.org/10.62441/nano-ntp.v20iS1.61 .
Article Google Scholar
Zain M, Ma H, Chaudhary S, Nuruzaman M, Azeem I, Mehmood F, Sun C. Nanotechnology in precision agriculture: advancing towards sustainable crop production. Plant Physiol Biochem. 2023. https://doi.org/10.1016/j.plaphy.2023.108244 .
Article PubMed Google Scholar
Jasmani L, Rusli R, Khadiran T, Jalil R, Dnan S. Application of nanotechnology in wood-based products industry: a review. Nanoscale Res Lett. 2020;15:1–31. https://doi.org/10.1186/s11671-020-03438-2 .
Alabdallah NM, Irshad MA, Rizwan M, Nawaz R, Inam A, Mohsin M, Ali S. Synthesis, characterization, and antifungal potential of titanium dioxide nanoparticles against fungal disease ( Ustilago tritici ) of wheat (Triticum aestivum m L.). Environ Res. 2023. https://doi.org/10.1016/j.envres.2023.115852 .
Chen F, Li Y, Irshad MA, Hussain A, Nawaz R, Qayyum MF, Ali S. Effect of titanium dioxide nanoparticles and co-composted biochar on growth and Cd uptake by wheat plants: a field study. Environ Res. 2023. https://doi.org/10.1016/j.envres.2023.116057 .
Article PubMed PubMed Central Google Scholar
Irshad MA, Nawaz R, Ur Rehman MZ, Adrees M, Rizwan M, Ali S, Tasleem S. Synthesis, characterization and advanced sustainable applications of titanium dioxide nanoparticles: a review. Ecotoxicol Environ Saf. 2021;212: 111978. https://doi.org/10.1016/j.ecoenv.2021.111978 .
Article CAS PubMed Google Scholar
Wang SS, Zhao MY, Zhou M, Li YCC, Wang J, Gao B. Biochar-supported nZVI (nZVI/BC) for contaminant removal from soil and water: a critical review. J Hazard Mater. 2019;373:820–34. https://doi.org/10.1016/j.jhazmat.2019.03.080 .
Santos CS, Gabriel B, Blanchy M, Menes O, García D, Blanco M, Neto V. Industrial applications of nanoparticles–a prospective overview. Mater Today Proc. 2015;2(1):456–65. https://doi.org/10.1016/j.matpr.2015.04.056 .
Korde P, Ghotekar S, Pagar T, Pansambal S, Oza R, Mane D. Plant extract assisted eco-benevolent synthesis of selenium nanoparticles-a review on plant parts involved, characterization and their recent applications. J Chem Rev. 2020;2(3):157–68. https://doi.org/10.33945/SAMI/JCR.2020.3.3 .
Article CAS Google Scholar
Pansambal S, Oza R, Borgave S, Chauhan A, Bardapurkar P, Vyas S, Ghotekar S. Bioengineered cerium oxide (CeO2) nanoparticles and their diverse applications: a review. Appl Nanosci. 2022. https://doi.org/10.1007/s13204-022-02574-8 .
Matussin S, Harunsani MH, Tan AL, Khan MM. Plantextract-mediated SnO2 nanoparticles: synthesis and applications. ACS Sustain Chemi Eng. 2020;8(8):3040–54. https://doi.org/10.1021/acssuschemeng.9b06398 .
Kashid Y, Ghotekar S, Bilal M, Pansambal S, Oza R, Varma RS, Mane D. Bio-inspired sustainable synthesis of silver chloride nanoparticles and their prominent applications. J Indian Chem Soc. 2022. https://doi.org/10.1016/j.jics.2021.100335 .
Kaur M, Gautam A, Guleria P, Singh K, Kumar V. Green synthesis of metal nanoparticles and their environmental applications. Curr Opin Environ Sci Health. 2022. https://doi.org/10.1016/j.coesh.2022.100390 .
Jeevanandam J, Kiew SF, Boakye-Ansah S, Lau SY, Barhoum A, Danquah MK. Rodrigues J Green approaches for the synthesis of metal and metal oxide nanoparticles using microbial and plant extracts. Nanoscale. 2022;14(7):2534–71. https://doi.org/10.1039/D1NR08144F .
Widatalla HA, Yassin LF, Alrasheid AA, Ahmed SAR, Widdatallah MO, Eltilib SH, Mohamed AA. Green synthesis of silver nanoparticles using green tea leaf extract, characterization and evaluation of antimicrobial activity. Nanoscale Adv. 2022;4(3):911–5. https://doi.org/10.1039/D1NA00509J .
Article CAS PubMed PubMed Central Google Scholar
Ye L, Cao Z, Liu X, Cui Z, Li Z, Liang Y, Wu S. Noble metal-based nanomaterials as antibacterial agents. J Alloys Compds. 2022;904: 164091. https://doi.org/10.1016/j.jallcom.2022.164091 .
Vankudoth S, Dharavath S, Veera S, Maduru N, Chada R, Chirumamilla P, Taduri S. Green synthesis, characterization, photoluminescence and biological studies of silver nanoparticles from the leaf extract of Muntingia calabura . Biochem Biophys Res Commun. 2022;630:143–50. https://doi.org/10.1016/j.bbrc.2022.09.054 .
Brar KK, Magdouli S, Othmani A, Ghanei J, Narisetty V, Sindhu R, Pandey A. Green route for recycling of low-cost waste resources for the biosynthesis of nanoparticles (NPs) and nanomaterials (NMs)-a review. Environ Res. 2022;207: 112202. https://doi.org/10.1016/j.envres.2021.112202 .
Parmar M, Sanyal M. Extensive study on plant mediated green synthesis of metal nanoparticles and their application for degradation of cationic and anionic dyes. Environ Nanotechnol Monit Manag. 2022;17: 100624. https://doi.org/10.1016/j.enmm.2021.100624 .
Gholami-Shabani M, Gholami-Shabani Z, Shams-Ghahfarokhi M, Jamzivar F, Razzaghi-Abyaneh M. Green nanotechnology: biomimetic synthesis of metal nanoparticles using plants and their application in agriculture and forestry. Nanotechnol Agric Paradigm. 2017. https://doi.org/10.1007/978-981-10-4573-8_8 .
Nazir R, Ayub Y, Tahir L. Green-nanotechnology for precision and sustainable agriculture. Biogenic Nanoparticles Agro Ecosyst. 2020. https://doi.org/10.1007/978-981-15-2985-6_18 .
Husen A, Bachheti RK, Bachheti A, editors. Current trends in green nano-emulsions: food, agriculture and biomedical sectors. Springer; 2023.
Google Scholar
Kashyap PL, Xiang X, Heiden P. Chitosan nanoparticle-based delivery systems for sustainable agriculture. J Biol Macromol. 2015;77:36–51. https://doi.org/10.1016/j.ijbiomac.2015.02.039 .
Jamdagni P, Khatri P, Rana JS. Green synthesis of zinc oxide nanoparticles using flower extract of Nyctanthes arbor-tristis and their antifungal activity. J King Saud Univ Sci. 2018;30:168–75. https://doi.org/10.1016/j.jksus.2016.10.002 .
Jamdagni P, Rana JS, Khatri P. Comparative study of antifungal effect of green and chemically synthesised silver nanoparticles in combination with carbendazim, mancozeb, and thiram. IET Nanobiotechnol. 2018;12:1102–7.
Jamdagni P, Rana JS, Khatri P, Nehra K. Comparative account of antifungal activity of green and chemically synthesized zinc oxide nanoparticles in combination with agricultural fungicides. IJND. 2018;9:198–208.
CAS Google Scholar
Shinde BH, Inamdar SN, Nalawade SA, Chaudhari SB. A systematic review on antifungal and insecticidal applications of biosynthesized metal nanoparticles. Mater Today Proc. 2023;73:412–7.
Kashyap PL, Rai P, Srivastava AK, Kumar S. Trichoderma for climate resilient agriculture. World J Microb Biotechnol. 2017;33:1–18. https://doi.org/10.1007/s11274-017-2319-1 .
Kaleem M, Minhas LA, Hashmi MZ, Farooqi HMU, Waqar R, Kamal K, Mumtaz AS. Biogenic synthesis of iron oxide nanoparticles and experimental modeling studies on the removal of heavy metals from wastewater. J J Saudi Chem Soc. 2024;28(1): 101777. https://doi.org/10.1016/j.jscs.2023.101777 .
Masood N, Irshad MA, Nawaz R, Abbas T, Abdel-Maksoud MA, AlQahtani WH, Abeed AH. Green synthesis, characterization, and adsorption of chromium and cadmium from wastewater using cerium oxide nanoparticles; reaction kinetics study. J Mol Struct. 2023;1294: 136563. https://doi.org/10.1016/j.molstruc.2023.136563 .
Irshad MA, Shakoor MB, Nawaz R, Yasmeen T, Arif MS, Rizwan M, Ali S. Green and eco-friendly synthesis of TiO2 nanoparticles and their application for removal of cadmium from wastewater: Reaction kinetics study (ZPC). Z Phys Chem. 2022;236(5):637–57. https://doi.org/10.1515/zpch-2021-3171 .
Irshad MA, Nawaz R, Wojciechowska E, Mohsin M, Nawrot N, Nasim I, Hussain F. Application of nanomaterials for cadmium adsorption for sustainable treatment of wastewater: a review. Wat Air Soil Poll. 2023;234(1):54. https://doi.org/10.1007/s11270-023-06064-7 .
Irshad MA, Sattar S, Nawaz R, Al-Hussain SA, Rizwan M, Bukhari A, Zaki ME. Enhancing chromium removal and recovery from industrial wastewater using sustainable and efficient nanomaterial: a review. Ecotoxicol Environ Saf. 2023;263(115231):10–1016.
Irshad MA, Shakoor MB, Ali S, Nawaz R, Rizwan M. Synthesis and application of titanium dioxide nanoparticles for removal of cadmium from wastewater: kinetic and equilibrium study. Wat Air Soil Poll. 2019;230(12):278. https://doi.org/10.1007/s11270-019-4321-8 .
Gangwar J, Kadanthottu Sebastian J, Puthukulangara Jaison J, Kurian JT. Nano-technological interventions in crop production—a review. Physiol Mol Biol Plants. 2023;29:93–107.
Balusamy SR, Joshi AS, Perumalsamy H, Mijakovic I, Singh P. Advancing sustainable agriculture: a critical review of smart and eco-friendly nanomaterial applications. J Nanobiotechnol. 2023;21:372.
Hazarika A, Yadav M, Yadav DK, Yadav HS. An overview of the role of nanoparticles in sustainable agriculture. ISBAB. 2022;43: 102399.
Singh RP, Handa R, Manchanda G. Nanoparticles in sustainable agriculture: an emerging opportunity. JCR. 2021;329:1234–48.
Enerijiofi KE, Musa SI, Igiebor FA, Lawani M, Chuka NE, Odozi EB, Ikhajiagbe B. Nanotechnological approaches for enhanced microbial activities, sustainable agriculture, and the environment. Sustain Agric Nanotechnol Biotechnol Crop Prod Protect. 2024. https://doi.org/10.1515/9783111234694-009 .
Behl K, Jaiswal P, Pabbi S. Recent advances in microbial and nano-formulations for effective delivery and agriculture sustainability. Biocatal Agric Biotechnol. 2024. https://doi.org/10.1016/j.bcab.2024.103180 .
Tripathi YC, Singh S, Anjum N, Srivastava KK. Antifungal activity of Capparis decidua extracts against seed borne pathogenic fungi. WJPPS. 2015;4:1500–12.
Ahmed HE, Iqbal Y, Aziz MH, Atif M, Batool Z, Hanif A, Ahmad H. Green synthesis of CeO2 nanoparticles from the Abelmoschus esculentus extract: evaluation of antioxidant, anticancer, antibacterial, and wound-healing activities. Molecules. 2021;26(15):4659. https://doi.org/10.3390/molecules26154659 .
Mosa MA, Youssef K, Hamed SF, Hashim AF. Antifungal activity of eco-safe nanoemulsions based on Nigella sativa oil against Penicillium verrucosum infecting maize seeds: Biochemical and physiological traits. Front Microbiol. 2023;13:1108733. https://doi.org/10.3389/fmicb.2022.1108733 .
Moore-Neibel K, Gerber C, Patel J, Friedman M, Ravishankar S. Antimicrobial activity of lemongrass oil against Salmonella enterica on organic leafy greens. J Appl Microbiol. 2012;112(3):485–92. https://doi.org/10.1111/j.1365-2672.2011.05222.x .
González-Fernández R, Prats E, Jorrín-Novo JV. Proteomics of plant pathogenic fungi. Biomed Res Int. 2010;1: 932527. https://doi.org/10.1155/2010/932527 .
Rajiv P, Rajeshwari S, Venckatesh R. Bio-Fabrication of zinc oxide nanoparticles using leaf extract of Parthenium hysterophorus L and its size-dependent antifungal activity against plant fungal pathogens. Spectrochimica Acta Part A Spectrochim Acta A Mol Biomol Spectrosc. 2013;112:384–7. https://doi.org/10.1016/j.saa.2013.04.072 .
Senthilkumar SR, Sivakumar T. Green tea (Camellia sinensis) mediated synthesis of zinc oxide (ZnO) nanoparticles and studies on their antimicrobial activities. Int J Pharm Pharm Sci. 2013;6(6):461–5.
Elsherbiny AS, Galal A, Ghoneem KM, Salahuddin NA. Graphene oxide-based nanocomposites for outstanding eco-friendly antifungal potential against tomato phytopathogens. Biomater Adv. 2024;2024(160): 213863. https://doi.org/10.1016/j.bioadv.2024.213863 .
Krishnaraj C, Ramachandran R, Mohan K, Kalaichelvan P. Optimization for rapid synthesis of silver nanoparticles and its effect on phytopathogenic fungi. Spectrochim Acta Part A Mol Biomol Spectrosc. 2012;93:95–9. https://doi.org/10.1016/j.saa.2012.03.002 .
Deshmukh SP, Patil SM, Mullani SB, Delekar SD. Silver nanoparticles as an effective disinfectant: a review. Mater Eng Res. 2019;97:954–65. https://doi.org/10.1016/j.msec.2018.12.102 .
Singh A, Agrawal M, Marshall FM. The role of organic vs inorganic fertilizers in reducing phytoavailability of heavy metals in a wastewater-irrigated area. Ecol Eng. 2010;36(12):1733–40. https://doi.org/10.1016/j.ecoleng.2010.07.021 .
Vargas Hernández R, Alvarez Lemus MA, De la Rosa GS, López González R, Quintana P, García Zaleta D, Gómez CS. Antifungal activity of ZnO nanoparticles synthesized from Eichhornia crassipes extract for construction applications. Nanomaterials. 2024;14(12):1007. https://doi.org/10.3390/nano14121007 .
Stałanowska K, Szablińska-Piernik J, Pszczółkowska A, Railean V, Wasicki M, Pomastowski P, Okorski A. Antifungal Properties of Bio-AgNPs against D . pinodes and F . avenaceum Infection of Pea ( Pisum sativum L.) seedlings. Int J Mol Sci. 2024;25(8):4525. https://doi.org/10.3390/ijms25084525 .
Ullah I, Rauf A, Khalil AA, Luqman M, Islam MR, Hemeg HA, Quradha MM. Peganum harmala L. extract-based Gold (Au) and Silver (Ag) nanoparticles (NPs): green synthesis, characterization, and assessment of antibacterial and antifungal properties. Food Sci Nutr. 2024. https://doi.org/10.1002/fsn3.4112 .
Lazcano-Ramírez HG, Garza-García JJ, Hernández-Díaz JA, León-Morales JM, Macías-Sandoval AS, García-Morales S. Antifungal activity of selenium nanoparticles obtained by plant-mediated synthesis. Antibiotics. 2023;12(1):115. https://doi.org/10.3390/antibiotics12010115 .
Alotaibi MO, Alotaibi NM, Ghoneim AM, Ul Ain N, Irshad MA, Nawaz R, Ali S. Effect of green synthesized cerium oxide nanoparticles on fungal disease of wheat plants: a field study. Chemosphere. 2023;339: 139731.
Rathi VH, Jeice AR, Jayakumar K. Green synthesis of Ag/CuO and Ag/TiO2 nanoparticles for enhanced photocatalytic dye degradation, antibacterial, and antifungal properties. Appl Surf Sci. 2023;18: 100476.
Ghorbani M, Tajik H, Moradi M, Molaei R, Alizadeh A. One-pot microbial approach to synthesize carbon dots from baker’s yeast-derived compounds for the preparation of antimicrobial membrane. Journal of J Environ Chem Eng. 2022;10(3): 107525.
Shahbaz M, Fatima N, Mashwani ZUR, Akram A, Haq EU, Mehak A, Pérez de la Lastra JM. Effect of phytosynthesized selenium and cerium oxide nanoparticles on wheat ( Triticum aestivum L.) against stripe rust disease. Molecules. 2022;27(23):8149.
Al-Otibi F, Perveen K, Al-Saif NA, Alharbi RI, Bokhari NA, Albasher G, Al-Mosa MA. Biosynthesis of silver nanoparticles using Malva parviflora and their antifungal activity. Saudi J Biol Sci. 2021;28(4):2229–35.
Ejaz H, Tariq M, Dawar S. Antifungal activity of selected halophytes against root pathogenic fungi. Int J Biol Biotechnol. 2021;18(1):113–8.
Yassin MA, Elgorban AM, El-Samawaty AERM, Almunqedhi BM. Biosynthesis of silver nanoparticles using Penicillium verrucosum and analysis of their antifungal activity. Saudi J Biol Sci. 2021;28:2123–7.
Dawoud TM, Yassin MA, El-Samawaty ARM, Elgorban AM. Silver nanoparticles synthesized by Nigrospora oryzae showed antifungal activity. Saudi J Biol Sci. 2021;28(3):1847–52.
Hasanin M, Al Abboud MA, Alawlaqi MM, Abdelghany TM, Hashem AH. Ecofriendly synthesis of biosynthesized copper nanoparticles with starch-based nanocomposite: antimicrobial, antioxidant, and anticancer activities. Biol Trace Elem Res. 2022;200:1–14.
Mali SC, Dhaka A, Githala CK, Trivedi R. Green synthesis of copper nanoparticles using Celastrus paniculatus Willd. Leaf extract and their photocatalytic and antifungal properties. Biotechnol Rep. 2020;27: e00518.
Win TT, Khan S, Fu P. Fungus—( Alternaria sp.) Mediated silver nanoparticles synthesis, characterization, and screening of antifungal activity against some phytopathogens. J Nanotechnol. 2020;2020:8828878.
Nguyen DH, Lee JS, Park KD, Ching YC, Nguyen XT, Phan VG, Hoang Thi TT. Green silver nanoparticles formed by Phyllanthus urinaria , Pouzolzia zeylanica and Scoparia dulcis leaf extracts and the antifungal activity. Nanomaterials. 2020;10(3):542.
Jebril S, Ben Jenana RK, Dridi C. Green synthesis of silver nanoparticles using Melia azedarach leaf extract and their antifungal activities: in vitro and in vivo. Mater Chem Phys. 2020;248: 122898.
Sahayaraj K, Balasubramanyam G, Chavali M. Green synthesis of silver nanoparticles using dry leaf aqueous extract of Pongamia glabra Vent (Fab) characterization and phytofungicidal activity. Environ Nanotechnol. 2020;14: 100349.
Ediz E, Kurtay G, Karaca B, Büyük I, Gökdemir F, Aras S. Green synthesis of silver nanoparticles from Phaseolus vulgaris L. extracts and investigation of their antifungal activities. Hacet J Biol Chem. 2020;49:11–23.
Kora AJ, Mounika J, Jagadeeshwar R. Rice leaf extract synthesized silver nanoparticles. An in vitro fungicidal evaluation against Rhizoctonia solani , the causative agent of sheath blight disease in rice. Fung Biol. 2020;124(7):671–81.
Irshad MA, Nawaz R, Ur Rehman MZ, Imran M, Ahmad J, Ahmad S, Ali S. Synthesis and characterization of titanium dioxide nanoparticles by chemical and green methods and their antifungal activities against wheat rust. Chemosphere. 2020;258: 127352.
Nguyen DH, Vo TNN, Nguyen NT, Ching YC, Thi TTH. Comparison of biogenic silver nanoparticles formed by Momordica charantia and Psidium guajava leaf extract and antifungal evaluation. PLoS ONE. 2020;15: e0239360.
Ahmad I, Khan SA, Naseer A, Nazir A. Green synthesized silver nanoparticles from Phoenix dactylifera synergistically interact with bioactive extract of Punica granatum against bacterial virulence and biofilm development. Microb Pathog. 2024;192: 106708.
El-Saadony MT, Saad AM, Najjar AA, Alzahrani SO, Alkhatib FM, Shafi ME, Hassan MA. The use of biological selenium nanoparticles to suppress Triticum aestivum L. crown and root rot diseases induced by Fusarium species and improve yield under drought and heat stress. Saudi J Biol Sci. 2021;28(8):4461–71.
Xiang S, Ma X, Shi H, Ma T, Tian C, Chen Y, Chen H, Chen X, Luo K, Cai L, et al. Green synthesis of an alginate-coated silver nanoparticle shows high antifungal activity by enhancing its cell membrane penetrating ability. ACS Appl Bio Mater. 2019;2:4087–96.
Pariona N, Mtz-Enriquez AI, Sánchez-Rangel D, Carrión G, Paraguay-Delgado F, Rosas-Saito G. Green-synthesized copper nanoparticles as a potential antifungal against plant pathogens. RSC Adv. 2019;9:18835–43.
Pham ND, Duong MM, Le MV, Hoang HA, Pham LK. Preparation and characterization of antifungal colloidal copper nanoparticles and their antifungal activity against Fusarium oxysporum and Phytophthora capsici . C R Chim. 2019;22:786–93.
Irshad MS, Aziz MH, Fatima M, Rehman SU, Idrees M, Rana S, Huang Q. Green synthesis, cytotoxicity antioxidant and photocatalytic activity of CeO 2 nanoparticles mediated via orange peel extract (OPE). Mater Res Expr. 2019;6(9):0950a4.
Prittesh K, Heena B, Rutvi B, Sangeeta J, Krunal M. Synthesis and characterisation of silver nanoparticles using withania somnifera and antifungal effect against Fusarium solani . Int J Plant Soil Sci. 2018;25:1–6.
Asghar MA, Zahir E, Shahid ZM, Khan MN, Asghar MA, Iqbal J, Walker G. (Iron, copper and silver nanoparticles Green synthesis using green and black tea leaves extracts and evaluation of antibacterial antifungal and aflatoxin B1 adsorption activity. Lwt. 2018;90:98–107.
Valsalam S, Agastian P, Arasu MV, Al-Dhabi NA, Ghilan AKM, Kaviyarasu K, Arokiyaraj S. Rapid biosynthesis and characterization of silver nanoparticles from the leaf extract of Tropaeolum majus L. and its enhanced in-vitro antibacterial antifungal antioxidant and anticancer properties. J Photochem Photobiol, B. 2019;191:65–74.
Elamawi RM, Al-Harbi RE, Hendi ZA. Biosynthesis and characterization of silver nanoparticles using Trichoderma longibrachiatum and their effect on phytopathogenic fungi. Egypt J Biol Pest Control. 2018;28:28.
Elshahawy I, Abouelnasr HM, Lashin SM, Darwesh OM. First report of Pythium aphanidermatum infecting tomato in Egypt and its control using biogenic silver nanoparticles. JPPR. 2018;58(2):137–51.
Vijayabharathi R, Sathy A, Gopalakrishnan S. Extracellular biosynthesis of silver nanoparticles using Streptomyces griseoplanus SAI-25 and its antifungal activity against Macrophomina phaseolina, the charcoal rot pathogen of sorghum. Biocatal Agric Biotechnol. 2018;14:166–71.
Rajeshkumar S, Rinitha G. Nanostructural characterization of antimicrobial and antioxidant copper nanoparticles synthesized using novel Persea americana seeds. OpenNano. 2018;3:18–27.
Huang W. Optimized biosynthesis and antifungal activity of Osmanthus fragrans leaf extract-mediated silver nanoparticles. Int J Agric Biol. 2017;19:668–72.
Abkhoo J, Panjehkeh N. Evaluation of antifungal activity of silver nanoparticles on Fusarium oxysporum. Int J Infect. 2017;4(2): e41126.
Jagana D, Hegde YR, Lella R. Green nanoparticles—a novel approach for the management of banana anthracnose caused by Colletotrichum musae . Int J Curr Microbiol Appl Sci. 2017;6:1749–56.
Sukhwal A, Jain D, Joshi A, Rawal P, Kushwaha HS. Biosynthesised silver nanoparticles using aqueous leaf extract of synthesized by the fungus aspergillus FOETIDUS MTCC8876. Dig J Nanomater Biostruct. 2013;8:197–205.
Bahrami-Teimoori B, Nikparast Y, Hojatianfar M, Akhlaghi M, Ghorbani R, Pourianfar HR. Characterisation and antifungal activity of silver nanoparticles biologically synthesised by Amaranthus retroflexus leaf extract. J Exp Nanosci. 2017;12:129–39.
Madbouly AK, Abdel-Aziz MS. Abdel-Wahhab MA Biosynthesis of nanosilver using Chaetomium globosum and its application to control Fusarium wilt of tomato in the greenhouse. IET Nanobiotechnol. 2017;11(6):702–8.
Article PubMed Central Google Scholar
Oloyede AR, Ayedun EO, Sonde OI, Akinduti PA. Investigation of antifungal activity of green-synthesized silver nanoparticles on phytopathogenic fungi. NJM. 2016;30:3323–8.
Ismail AW, Sidkey N, Arafa R, Fathy R, El-Batal A. Evaluation of in vitro antifungal activity of silver and selenium nanoparticles against Alternaria solani caused early blight disease on potato. Br Biotechnol J. 2016;12(3):1–11.
Fernández JG, Fernández-Baldo MA, Berni E, Camí G, Durán N, Raba J, Sanz MI. Production of silver nanoparticles Fungi. Adv Anim Vet Sci. 2019;7:238–49.
Elgorban AM, Aref SM, Seham SM, Elhindi KM, Bahkali AH, Sayed SR, Manal MA. Extracellular synthesis of silver nanoparticles using Aspergillus versicolor and evaluation of their activity on plant pathogenic fungi. Mycosphere. 2016;7:844–52. https://doi.org/10.5943/mycosphere/7/6/15 .
Jafari A, Pourakbar L, Farhadi K, Mohamadgolizad L, Goosta Y. Biological synthesis of silver nanoparticles and evaluation of antibacterial and antifungal properties of silver and copper nanoparticles. Turk J Biol. 2015;39:556–61. https://doi.org/10.3906/biy-1406-81 .
Velmurugan P, Sivakumar S, Young-Chae S, Seong-Ho J, Pyoung-In Y, Jeong-Min S, Sung-Chul H. Synthesis and characterization comparison of peanut shell extract silver nanoparticles with commercial silver nanoparticles and their antifungal activity. J Ind Eng Chem. 2015;31:51–4. https://doi.org/10.1016/j.jiec.2015.06.031 .
Abd El-Aziz ARM, Al-Othman MR, Mahmoud MA, Metwaly HA. Biosynthesis of silver nanoparticles using fusarium against Rhizoctonia solani , the causative agent of sheath blight disease in rice. Fungal Biol. 2020;124:671–81.
Bholay AD, Nalawade PM, Borkhataria BV. Fungicidal potential of biosynthesized silver nanoparticles against phytopathogens and potentiation of fluconazole. World J Pharm Res. 2013;1:12–5.
Al-Othman MR, Abd El-Aziz ARM, Mahmoud MA, Eifan SA, El-Shikh MS, Majrashi M. Application of silver nanoparticles as antifungal and antiaflatoxin B1 produced by Aspergillus flavus . Dig J Nanomater Bios. 2014;9(1):151–7.
Elamawi RM, Al-Harbi RE. Effect of biosynthesized silver nanoparticles on Fusarium oxysporum fungus the cause of seed rot disease of faba bean, tomato and barley. J Plant Prot Pathol. 2014;5:225–37. https://doi.org/10.21608/JPPP.2014.87901 .
Roy S, Mukherjee T, Chakraborty S, Das TK. Biosynthesis, characterisation & antifungal activity of silver nanoparticles synthesized by the fungus Aspergillus foetidus MTCC8876. DJNB. 2013;8(1):197–205.
Aguilar-Méndez MA, San Martín-Martínez E, Ortega-Arroyo L, Cobián-Portillo G, Sánchez-Espíndola E. Synthesis and characterization of silver nanoparticles: effect on phytopathogen Colletotrichum gloesporioides . J Nanoparticle Res. 2011;13:2525–32. https://doi.org/10.1007/s11051-010-0145-6 .
Lopez-Lima D, Mtz-Enriquez AI, Carrión G, Basurto-Cereceda S, Pariona N. The bifunctional role of copper nanoparticles in tomato: effective treatment for Fusarium wilt and plant growth promoter. Sci Hortic. 2010;277: 109810. https://doi.org/10.1016/j.scienta.2020.109810 .
Nargund VB, Vinay JU, Basavesha KN, Chikkanna S, Jahagirdar S, Patil RR. Green nanotechnology and its application in plant disease management. Plant Pathol J. 2021. https://doi.org/10.1007/978-981-15-6275-4_26 .
Tolaymat T, Genaidy A, Abdelraheem W, Dionysiou D, Andersen C. The effects of metallic engineered nanoparticles upon plant systems: an analytic examination of scientific evidence. Sci Total Environ. 2017;579:93–106. https://doi.org/10.1016/j.scitotenv.2016.10.229 .
Kumar S, Sachdeva S, Bhat KV, Vats S. Plant responses to drought stress: physiological, biochemical and molecular basis. In Biotic and abiotic stress tolerance in plants. 2018; 1–25. https://doi.org/10.1007/978-981-10-9029-5_1
Shende S, Rajput VD, Gade A, Minkina T, Fedorov Y, Sushkova S, Boldyreva V. Metal-based green synthesized nanoparticles: boon for sustainable agriculture and food security. Nano Bioscience. 2021;21(1):44–54. https://doi.org/10.1109/TNB.2021.3089773 .
Ameena S, Rajesh N, Anjum SM, Khadri H, Riazunnisa K, Mohammed A, Kari ZA. Antioxidant, antibacterial, and anti-diabetic activity of green synthesized copper nanoparticles of Cocculus hirsutus (Menispermaceae). Appl Biochem Biotechnol. 2022;194(10):4424–38. https://doi.org/10.1007/s12010-022-03899-4 .
Kamis Y, Hashim A, Margarita R, Algothaymi MA, Abd-Elsalam KA. Fungicidal efficacy of chemically produced copper nanoparticles against Penicillium digitatum and Fusarium solani on citrus fruit. Philipp Agric Sci. 2017;100(1):69–78.
Arunachalam KD, Annamalai SK, Hari S. One-step green synthesis and characterization of leaf extract-mediated biocompatible silver and gold nanoparticles from Memecylon umbellatum . Int J Nanomed. 2013. https://doi.org/10.2147/IJN.S36670 .
Yuan X, An N, Zhu Z, Sun H, Zheng J, Jia M, Liu N. Hierarchically porous nitrogen-doped carbon materials as efficient adsorbents for removal of heavy metal ions. Process Saf Environ Prot. 2018;119:320–9. https://doi.org/10.1016/j.psep.2018.08.012 .
Wacławek S, Gončuková Z, Adach K, Fijałkowski M, Černík M. Green synthesis of gold nanoparticles using Artemisia dracunculus extract: control of the shape and size by varying synthesis conditions. Environ Sci Pollut Res Int. 2018;25:24210–9. https://doi.org/10.1007/s11356-018-2510-4 .
Anand K, Murugan V, Mohana Roopan S, Surendra TV, Chuturgoon AA, Muniyasamy S. Degradation treatment of 4-nitrophenol by Moringa oleifera synthesised GO-CeO 2 nanoparticles as catalyst. J Inorg Organomet Polym Mater. 2018;28:2241–8. https://doi.org/10.1007/s10904-018-0891-y .
Kumar V, Yadav SK. Characterisation of gold nanoparticles synthesised by leaf and seed extract of Syzygium cumini L. J Exp Nanosci. 2012;7(4):440–51.
Bhau BS, Ghosh S, Puri S, Borah B, Sarmah DK, Khan R. Green synthesis of gold nanoparticles from the leaf extract of Nepenthes khasiana and antimicrobial assay. Adv Mater Lett. 2015;6(1):55–8. https://doi.org/10.5185/amlett.2015.5609 .
Mittal D, Kaur G, Singh P, Yadav K, Ali SA. Nanoparticle-based sustainable agriculture and food science: recent advances and future outlook. Front nanotechnol. 2020;2020(2):10. https://doi.org/10.3389/fnano.2020.579954 .
Hussien NA. Antimicrobial potential of biosynthesized zinc oxide nanoparticles using banana peel and date seeds extracts. Sustainability. 2023;15(11):9048. https://doi.org/10.3390/su15119048 .
Castillo-Henríquez L, Alfaro-Aguilar K, Ugalde-Álvarez J, Vega-Fernández L, Montes Oca-Vásquez G, Vega-Baudrit JR. Green synthesis of gold and silver nanoparticles from plant extracts and their possible applications as antimicrobial agents in the agricultural area. Nanomaterials. 2020;10(9):1763. https://doi.org/10.3390/nano10091763 .
Azizi S, Ahmad MB, Namvar F, Mohamad R. Green biosynthesis and characterization of zinc oxide nanoparticles using brown marine macroalga Sargassum muticum aqueous extract. Mater Lett. 2014;116:275–7. https://doi.org/10.1016/j.matlet.2013.11.038 .
Khan MR, Rizvi TF. Nanotechnology: scope and application in plant disease management. Plant Patholol. 2014;13(3):214–31. https://doi.org/10.3923/ppj.2014.214.231 .
Nasrollahzadeh M, Atarod M, Sajjadi M, Sajadi SM, Issaabadi Z. Plant-mediated green synthesis of nanostructures: mechanisms, characterization, and applications. Interface Sci Technol. 2019;28:99–322. https://doi.org/10.1016/B978-0-12-813586-0.00006-7 .
Vijayaram S, Razafindralambo H, Sun YZ, Vasantharaj S, Ghafarifarsani H, Hoseinifar SH, Raeeszadeh M. Applications of green synthesized metal nanoparticles—a review. Biol Trace Elem Res. 2024;202:360–86.
Pandey S. Recent advances in green nanotechnology for environmental sustainability. J Clean Prod. 2018;194:1065–84.
Aithal PS, Aithal S. Green nanotechnology for environment and sustainability. IJANS. 2015;4:33–52.
Karn B, Wong D. Nanotechnology and the environment: applications and implications. Environ Sci Technol. 2013;47:10083–4.
Peralta-Videa JR, Huang Y, Parsons JG, Zhao L, Lopez-Moreno L, Hernandez-Viezcas JA, Gardea-Torresdey JL. Plant-based green synthesis of metallic nanoparticles: scientific curiosity or a realistic alternative to chemical synthesis? Nanotechnol Environ Eng. 2016;1:1–29.
Nabi G, Raza W, Tahir M. Green synthesis of TiO2 nanoparticle using cinnamon powder extract and the study of optical properties. J Inorg Organomet Polym Mater. 2019. https://doi.org/10.1007/s10904-019-01248-3 .
Hong J, Wang C, Wagner DC, Gardea-Torresdey JL, He F, Rico CM. Foliar application of nanoparticles: mechanisms of absorption, transfer, and multiple impacts. Environ Sci Nano. 2021;8:1196–210.
Nasrollahi A, Pourshamsian KH, Mansourkiaee P. Antifungal activity of silver nanoparticles on some of fungi; 2011
Paulo CS, Vidal M, Ferreira LS. Antifungal nanoparticles and surfaces. Biomacromol. 2010;11:2810–7.
Khan ST, Ahmad J, Ahamed M, Jousset A. Sub-lethal doses of widespread nanoparticles promote antifungal activity in Pseudomonas protegens CHA0. Sci Total Environ. 2018;627:658–62.
Khan M, Wali R, Mashwani ZUR, Raja NI, Ullah R, Bari A, Sohail A. Nanowarriors from mentha: unleashing nature’s antimicrobial arsenal with cerium oxide nanoparticles. ACS Omega. 2024;9(13):15449–62. https://doi.org/10.1021/acsomega.4c00236 .
Hosseinzadeh F, Tabesh H, Farzaneh F. Nano drug delivery platform based on thermosensitive PEG-PCL hydrogel encapsulated in silver-bearing micelles and its antifungal activity investigation against vaginal candidiasis. Front Mater Sci. 2023;10:1210542. https://doi.org/10.3389/fmats.2023.1210542 .
Auyeung A, Casillas-Santana MA, Martinez-Castanon GA, Slavin YN, Zhao W, Asnis J, Bach H. Effective control of molds using a combination of nanoparticles. PLoS ONE. 2017;12(1): e0169940. https://doi.org/10.1371/journal.pone.0169940 .
Rosa-García E, Olalde-Hernández MJ, Irigoyen-Camacho ME, Mondragón-Padilla A, Mendoza-Juache A, Sánchez-Vargas LO. Antifungal susceptibility of oral isolates of Candida species from chronic kidney disease patients on chronic dialysis. JMM. 2020;30(3): 101009. https://doi.org/10.1016/j.mycmed.2020.101009 .
Bhavyasree PG, Xavier TS. Green synthesised copper and copper oxide-based nanomaterials using plant extracts and their application in antimicrobial activity. GRGSC. 2022;5: 100249. https://doi.org/10.1016/j.crgsc.2021.100249 .
Abd-Elsalam KA, Vasilkov AY, Said-Galiev EE, Rubina MS, Khokhlov AR, Naumkin AV, Alghuthaymi MA. Bimetallic blends and chitosan nanocomposites: Novel antifungal agents against cotton seedling damping-off. Eur J Plant Pathol. 2018;151:57–72. https://doi.org/10.1007/s10658-017-1349-8 .
Youssef GA, Mohamed AS. In-vitro antifungal activity of eco-friendly essential oils against pathogenic seed borne fungi. EJBO. 2020;60(2):381–93. https://doi.org/10.21608/EJBO.2019.17672.1362 .
Malode GP, Parbat AY, Shaikh AR, Panchale WA, Manwar JV, Bakal RL. Phytochemistry, pharmacology and botanical aspects of Murraya Koenigii in the search for molecules with bioactive potential-A review. GSCARR. 2021;6(3):143–55. https://doi.org/10.30574/gscarr.2021.6.3.0055 .
Fernandes KE, Carter DA. The antifungal activity of lactoferrin and its derived peptides: mechanisms of action and synergy with drugs against fungal pathogens. Front microbiol. 2017;8:2. https://doi.org/10.3389/fmicb.2017.00002 .
Yesilay G, Dos Santos OAL, Hazeem LJ, Backx BP, Kamel AH, Bououdina M. Impact of pathogenic bacterial communities present in wastewater on aquatic organisms: application of nanomaterials for the removal of these pathogens. Aquat Toxicol. 2023;261: 106620. https://doi.org/10.1016/j.aquatox.2023.106620 .
Sánchez-López E, Gomes D, Esteruelas G, Bonilla L, Lopez-Machado AL, Galindo R, Souto EB. Metal-based nanoparticles as antimicrobial agents: an overview. Nanomaterials. 2020;10:292. https://doi.org/10.3390/nano10020292 .
Zhang H, Lu L, Zhao X, Zhao S, Gu X, Du W, Wei H, Ji R, Zhao L. Metabolomics reveals the “invisible” responses of spinach plants exposed to CeO 2 nanoparticles. Environ sci technol. 2019;53:6007–17. https://doi.org/10.1021/acs.est.9b00593 .
Kuang Y, He X, Zhang Z, Li Y, Zhang H, Ma Y, Chai Z. Comparison study on the antibacterial activity of nano-or bulk-cerium oxide. JNN. 2011;11:4103–8. https://doi.org/10.1166/jnn.2011.3858 .
Gonzalez JM, Rodriguez CA, Agudelo M, Zuluaga AF, Vesga O. Antifungal pharmacodynamics: Latin America’s perspective. BJID. 2017;21:79–87. https://doi.org/10.1016/j.bjid.2016.09.009 .
Turan NB, Erkan HS, Engin GO, Bilgili MS. Nanoparticles in the aquatic environment: usage, properties, transformation and toxicity—a review. PSEP. 2019;130:238–49.
Tran TK, Nguyen MK, Lin C, Hoang TD, Nguyen TC, Lone AM, Nguyen DD. Review on fate, transport, toxicity and health risk of nanoparticles in natural ecosystems: emerging challenges in the modern age and solutions toward a sustainable environment. Sci Total Environ. 2023;912: 169331.
Gatoo MA, Naseem S, Arfat MY, Mahmood Dar A, Qasim K, Zubair S. Physicochemical properties of nanomaterials: implication in associated toxic manifestations. Biomed Res Int. 2014;1: 498420.
Lee S, Chen TT, Barber CL, Jordan MC, Murdock J, Desai S, Iruela-Arispe ML. Autocrine VEGF signaling is required for vascular homeostasis. Cell. 2007;130:691–703.
Chand R, Singh J. From green revolution to amrit kaal. Natl Inst Transf India. 2023;3:67–89.
Mushtaque MAR. Integration of wireless sensor networks, internet of things, artificial intelligence, and deep learning in smart agriculture: a comprehensive survey: integration of wireless sensor networks. Internet of Things JIICET. 2024;1:8–19.
Thamik H, Cabrera JDF, Wu J. The digital paradigm: unraveling the impact of artificial intelligence and internet of things on achieving sustainable development goals. AIoT. 2024;4:21–40. https://doi.org/10.1007/978-3-031-53433-1_2 .
Download references
Acknowledgements
The authors express their gratitude to the Deanship of Scientific Research, Imam Mohammad Ibn Saud Islamic University, Saudi Arabia to support this research work. The authors express sincere gratitude to the Department of Environmental Sciences, The University of Lahore, Pakistan for the provision of laboratory work and resources provided for this research.
This work was supported and funded by the Deanship of Scientific Research at Imam Mohammad Ibn Saud Islamic University (IMSIU).
Author information
Authors and affiliations.
Department of Environmental Sciences, The University of Lahore, Lahore, 54000, Pakistan
Muhammad Atif Irshad, Azhar Hussain, Iqra Nasim, Rab Nawaz & Shaheryar Azeem
Faculty of Engineering and Quantity Surveying, INTI International University, 71800, Nilai, Negeri Sembilan, Malaysia
Department of Chemistry, College of Science, Imam Mohammad Ibn Saud Islamic University (IMSIU), 11623, Riyadh, Saudi Arabia
Aamal A. Al-Mutairi, Sami A. Al-Hussain & Magdi E. A. Zaki
Department of Environmental Sciences, Government College University Faisalabad, Faisalabad, 38000, Pakistan
Muhammad Rizwan
Department of Chemistry, Government College University Faisalabad, Faisalabad, 38000, Pakistan
You can also search for this author in PubMed Google Scholar
Contributions
Muhammad Atif Irshad: conceptualization, supervision, writing—original draft, writing—review & editing. Azhar Hussain: resources, writing—review & editing. Iqra Nasim: writing—original draft, writing—review & editing. Rab Nawaz: conceptualization, writing—original draft. Aamal A. Al-Mutairi; data curation, writing—review & editing. Shaheryar Azeem: writing—original draft, visualization; Muhammad Rizwan; resources, writing—review & editing. Sami A. Al-Hussain; visualization, data curation. Writing—original draft; Ali Irfan: formal analysis (Literature Survey), visualization, funding acquisition, writing—review & editing. Magdi E. A. Zaki: funding acquisition, project administration, formal analysis (Literature Survey), writing—review & editing. All authors have read and agreed to the published version of the manuscript.
Corresponding authors
Correspondence to Rab Nawaz , Ali Irfan or Magdi E. A. Zaki .
Ethics declarations
Competing interests.
The authors declare no competing interests.
Additional information
Publisher's note.
Springer Nature remains neutral with regard to jurisdictional claims in published maps and institutional affiliations.
Rights and permissions
Open Access This article is licensed under a Creative Commons Attribution-NonCommercial-NoDerivatives 4.0 International License, which permits any non-commercial use, sharing, distribution and reproduction in any medium or format, as long as you give appropriate credit to the original author(s) and the source, provide a link to the Creative Commons licence, and indicate if you modified the licensed material. You do not have permission under this licence to share adapted material derived from this article or parts of it. The images or other third party material in this article are included in the article’s Creative Commons licence, unless indicated otherwise in a credit line to the material. If material is not included in the article’s Creative Commons licence and your intended use is not permitted by statutory regulation or exceeds the permitted use, you will need to obtain permission directly from the copyright holder. To view a copy of this licence, visit http://creativecommons.org/licenses/by-nc-nd/4.0/ .
Reprints and permissions
About this article
Cite this article.
Irshad, M.A., Hussain, A., Nasim, I. et al. Exploring the antifungal activities of green nanoparticles for sustainable agriculture: a research update. Chem. Biol. Technol. Agric. 11 , 133 (2024). https://doi.org/10.1186/s40538-024-00662-1
Download citation
Received : 19 July 2024
Accepted : 31 August 2024
Published : 11 September 2024
DOI : https://doi.org/10.1186/s40538-024-00662-1
Share this article
Anyone you share the following link with will be able to read this content:
Sorry, a shareable link is not currently available for this article.
Provided by the Springer Nature SharedIt content-sharing initiative
- Green nanotechnology
- Metallic NPs
- Antifungal activity
- Biotic stresses
- Sustainable agriculture
- Crop production

IMAGES
VIDEO
COMMENTS
Since the start of the agricultural revolution, the sector has been defined by research and innovation, which include technology development that is adopted throughout the value chain, comprehensive and inclusive of digital solutions. And this also includes the very important topic of ethical and safe practices - both for industry role ...
4. Conclusion. Research in agriculture helps in the development of new methods and techniques of farming, some of which are more efficient, cost-effective, and ecologically beneficial. These new techniques, if implemented in the right way, can help increase the productivity of a farm and lower the risk to crops.
This report contains a review of the literature on the role of agricultural research and development in fostering innovation and productivity in agriculture. The review seeks to clarify concepts and terminology used in the area, provide a critical assessment of approaches found in the literature, report main results, and draw inferences.
Accordingly, research in the field of agriculture needs to be reconsidered. In this part, ... have put forward a broader conception of "use" in evaluation research, which highlights the importance of considering the research "influence." These led to paradigmatic changes in attitudes toward the "use" of research findings, and ...
1. Introduction. Research has multiple impacts on society. In the light of the international discourse on grand societal challenges and sustainable development, the debate is reinforced about the role of research on economic growth, societal well-being, and environmental integrity ().Research impact assessment (RIA) is a key instrument to exploring this role ().
Transforming research into results for rural people. Agricultural research is essential for sustainable and inclusive agricultural development. Research generates new technologies and improved policies which are essential for small-scale farmers who face the interconnected challenges of climate change, land degradation, gender biases, hunger ...
A previous paper about the top 100 questions of importance to the future of global agriculture was published almost a decade ago, with contributors primarily comprising experts and representatives from agricultural organizations. 4 Our collection was intended for a broad community, including scientists, engineers, farmers, entrepreneurs ...
8. Conclusion. In going from lab to field, experiments have revolutionized a significant part of the research development economists do to understand how agriculture is and can be used for development. The approach has taken them to the field and engaged them into action research with development partners.
Abstract. Restructuring farmer-researcher relationships and addressing complexity and uncertainty through joint exploration are at the heart of On-Farm Experimentation (OFE). OFE describes new ...
The challenges facing global agriculture via population increase, climate change and dietary choices are unprecedented and urgent. In the context of declining public funding for research and development in agriculture (ag R&D), we highlight the historically high returns on such investments and outline an economic rationale to continue government involvement through support and policy ag R&D.
Global volatility of public agricultural R&D expenditure. Stuti Rawat, in Advances in Food Security and Sustainability, 2020. Abstract. Public investment in agricultural research and development (R&D) is important for global food security and environmental sustainability. Although public agricultural R&D projects are associated with high economic returns, they are characterized by long time ...
The domestication of agriculture is widely recognized as one of the most crucial technological adaptations for the transition of humanity from hunter-and-gatherer groups into early city-states and ...
The " USDA Science and Research Strategy, 2023-2026: Cultivating Scientific Innovation (PDF, 21.4 MB)" presents a near-term vision for transforming U.S. agriculture through science and innovation, and outlines USDA's highest scientific priorities. The S&RS is a call to action for USDA partners, stakeholders, and customers to join the ...
The great new feature of the modern progress in agriculture is. the rapid increase in the utilization of science and the results of. scientific research as a source of information and guidance in im- proving and perfecting agricultural methods. Research in agriculture may be divided into two main classes: strictly agricultural research, and ...
Two databases including yield, management, and weather data for maize (n = 17,013) and soybean (n = 24,848) involving US crop performance trials conducted in 28 states between 2016 to 2018 for ...
The Role of Research in Agriculture. people of widely different interests and will need more than ever enough alter. disciplines to cooperate closely. This natives and new ideas to be flexible and means that, perhaps, physicists and avoid the crises that come from de psychologists, seismologists and social pending too heavily on any one break.
Agricultural research seems to be the oldest form of organized research in the world. Agricultural research can be broadly defined as any research activity aimed at improving productivity and quality of crops by their genetic improvement, better plant protection, irrigation, storage methods, farm mechanization, efficient marketing, and a better management of resources.
Agriculture can help reduce poverty for 75% of the world's poor, who live in rural areas and work mainly in farming. It can raise incomes, improve food security and benefit the environment. The World Bank Group is a leading financier of agriculture, with $8.1 billion in new commitments in 2013.
Increasing the role of farmers in agricultural innovation can help them catch up—and will lead to better science in the process. Famines have killed tens of millions of people across the centuries. But as the global population rose from 2.5 billion people in 1950 to nearly eight billion today, mortality from famine was radically curtailed.
The world's 570 million farmers are arguably the most important stewards of the earth's land, water and biodiversity. Worldwide, farming uses around 40% of total land area, two-thirds of water withdrawals and 85% of water consumption today. This is up from around 7% of total land area back in the year 1700 when the population was less than ...
Increasing of agriculture products is of importance so that research and development in this branch considers as one of the most important infrastructure which develops production growth.
Both crops had an important role in early 19 th-century agriculture in the UK as a source of N, especially for wheat for bread that followed beans or clover in the Norfolk four-course arable rotation. From 1847, field beans were grown each year on Geescroft, and from 1849 attempts were made to grow clover continuously on a part of Hoosfield.
Agriculture also impacts economic development by contributing to the overall U.S. gross domestic product (GDP), directly and indirectly. It does so through farm production, forestry, fishing activities, textile mills and products, apparel and food and beverage sales, and service and manufacturing. Farm production.
WASHINGTON — Rep. Rodney Davis (IL-13), Chairman of the House Agriculture Committee's Subcommittee on Biotechnology, Horticulture, and Research, held a hearing to highlight the importance of agricultural research as part of the committee's hearing series on the next farm bill. Members heard from witnesses who stressed the important role research plays in ensuring that American […]
Moreover, research is also required to identify the best methods for applying green NPs for crop production and sustainable agriculture. Furthermore, research should be undertaken to establish the most cost-effective methods of making and deploying green nanoparticles at a large field size study where there is fungal attack that diminishes ...