
- General & Introductory Agriculture

Plant Biotechnology Journal
Print ISSN: 1467-7644
Online ISSN: 1467-7652
Impact Factor: 10.1
Society for Experimental Biology , Association of Applied Biologists
Edited By:Johnathan Napier

An official website of the United States government
Here's how you know
The .gov means it’s official. Federal government websites often end in .gov or .mil. Before sharing sensitive information, make sure you’re on a federal government site.
The site is secure. The https:// ensures that you are connecting to the official website and that any information you provide is encrypted and transmitted securely.
About Grants
The lifecycle of grants and cooperative agreements consists of four phases: Pre-Award, Award, Post-Award, and Close Out.
Access to Data
The National Institute of Food and Agriculture is committed to serving its stakeholders, Congress, and the public by using new technologies to advance greater openness.

Access Data Gateway
The Data Gateway enables users to find funding data, metrics, and information about research, education, and extension projects that have received grant awards from NIFA.
View Resources Page
This website houses a large volume of supporting materials. In this section, you can search the wide range of documents, videos, and other resources.

Featured Webinar
Second annual virtual grants support technical assistance workshop.
Check out this five-day workshop in March 2024 workshop, designed to help you learn about NIFA grants and resources for grants development and management.
The National Institute of Food and Agriculture provides leadership and funding for programs that advance agriculture-related sciences.

Plant Biotechnology
Plant biotechnology is a set of techniques used to adapt plants for specific needs or opportunities. Situations that combine multiple needs and opportunities are common.
For example, a single crop may be required to provide sustainable food and healthful nutrition, protection of the environment, and opportunities for jobs and income. Finding or developing suitable plants is typically a highly complex challenge.
Plant biotechnologies that assist in developing new varieties and traits include genetics and genomics , marker-assisted selection (MAS) , and transgenic (genetic engineered) crops. These biotechnologies allow researchers to detect and map genes, discover their functions, select for specific genes in genetic resources and breeding, and transfer genes for specific traits into plants where they are needed. NIFA funds research, training, and extension for developing and using biotechnologies for food and agriculture. Areas of work include, but not limited to:
- Genetic structures and mechanisms
- Methods for transgenic biotechnology (also known as genetic engineering)
- Identification of traits and genes that can contribute to national and global goals for agriculture
- Plant genome sequences; molecular markers, and bioinformatics
- Gene Editing/Genome Editing
- Synthetic Biology
Most public research on transgenic crops focuses in some way on two general objectives:
- Better understanding of all aspects of the transgenic/genetic engineering process, for enhancing efficiency, precision, and proper expression of the added genes or nucleic acid molecules.
- A wider range of useful and valuable traits, including complex traits.
Competitive Funding Programs in Plant Biotechnology
Agriculture and Food Research Initiative (AFRI) program
Biotechnology Risk assessment Grants (BRAG) program
Small Business Innovation Research (SBIR) program
Specialty Crop Research Initiative (SCRI) program
Examples of Biotechnology Risk Assessment Grant (BRAG) projects
Latest Updates
- Latest Funding Opportunities
- Latest Blogs
- Latest Impacts
funding opportunity
Mentoring at risk and rural youth, small business innovation research and small business technology transfer programs phase i, open data framework, growers helping growers avoid a devastating cranberry disease, more than a market, nifa invests $7.4m in data science for food and agricultural systems (a1541), nifa-funded research results in technological innovation that saves florida citrus industry, nifa-funded research helps reduce food loss, better berries, cold-tolerant sugarcane, precision breeding is making it possible, your feedback is important to us..

An official website of the United States government
The .gov means it’s official. Federal government websites often end in .gov or .mil. Before sharing sensitive information, make sure you’re on a federal government site.
The site is secure. The https:// ensures that you are connecting to the official website and that any information you provide is encrypted and transmitted securely.
- Publications
- Account settings
Preview improvements coming to the PMC website in October 2024. Learn More or Try it out now .
- Advanced Search
- Journal List
- J Genet Eng Biotechnol
- v.20; 2022 Dec

Bioinformatics approaches and applications in plant biotechnology
Yung cheng tan.
1 Division of Applied Biomedical Sciences and Biotechnology, School of Health Sciences, International Medical University, 126 Jalan Jalil Perkasa 19, Bukit Jalil, 57000 Kuala Lumpur, Malaysia
Asqwin Uthaya Kumar
2 School of Biosciences and Biotechnology, Faculty of Science and Technology, Universiti Kebangsaan Malaysia, 43600 Bangi, Malaysia
Ying Pei Wong
Anna pick kiong ling, associated data.
Not applicable.
In recent years, major advance in molecular biology and genomic technologies have led to an exponential growth in biological information. As the deluge of genomic information, there is a parallel growth in the demands of tools in the storage and management of data, and the development of software for analysis, visualization, modelling, and prediction of large data set.
Particularly in plant biotechnology, the amount of information has multiplied exponentially with a large number of databases available from many individual plant species. Efficient bioinformatics tools and methodologies are also developed to allow rapid genome sequence and the study of plant genome in the ‘omics’ approach. This review focuses on the various bioinformatic applications in plant biotechnology, and their advantages in improving the outcome in agriculture. The challenges or limitations faced in plant biotechnology in the aspect of bioinformatics approach that explained the low progression in plant genomics than in animal genomics are also reviewed and assessed.
There is a critical need for effective bioinformatic tools, which are able to provide longer reads with unbiased coverage in order to overcome the complexity of the plant’s genome. The advancement in bioinformatics is not only beneficial to the field of plant biotechnology and agriculture sectors, but will also contribute enormously to the future of humanity.
Over the past decades, the term ‘bioinformatics’ has become a buzzword in all areas of research in biological science. With the continuous development and advancement in molecular biology, the explosive growth of biological information required a more organized, computerized system to collect, store, manage, and analyse the vast amount of biological data generated in the experiments from all fields [ 1 ]. Bioinformatics, as a new emerging interdisciplinary field for the past few decades, has many tools and techniques that are essential for efficient sorting and organizing of biological data into databases [ 1 , 2 ]. Bioinformatics can be referred as a computer-based scientific field which applies mathematics, biology, and computer science to form into a single discipline for the analyses and interpretation of genomics and proteomics data [ 2 , 3 ]. In short, the main components of bioinformatics are (a) the collection and analysis of database and (b) the development of software tools and algorithm as a tool for interpretation of biological data [ 2 ]. Bioinformatics played a crucial role in many areas of biology as its applications provide various types of data, including nucleotide and amino acid sequences, protein domains and structure as well as expression patterns from various organisms [ 3 ]. Similarly, the field of plant biotechnology has also taken advantages of bioinformatics, which provides full genomic information of various plant species to allow for efficient exploration into plants as biological resource to humans [ 1 , 3 , 4 ]. The intention of this article is to describe some of the key concepts, tools, and its applications in bioinformatics that are relevant to plant biotechnologies. The current challenges and limitations for improvement and continuous development of bioinformatics in plant science are also described.
Applications of bioinformatics in plant biotechnology
The introduction of bioinformatics and computational biology into the area of plant biology is drastically accelerating scientific invention in life science. With the aid of sequencing technology, scientists in plant biology have revealed the genetic architecture of various plant and microorganism species, such as proteome, transcriptome, metabolome, and even their metabolic pathway [ 1 ]. Sequence analysis is the most fundamental approach to obtain the whole genome sequence such as DNA, RNA, and protein sequence from an organism’s genome in modern science. The sequencing of whole genome permits the determination of organization of different species and provides a starting point to understand their functionality. A complete sequence data consists of coding and non-coding regions, which can act as a necessary precursor for any functional gene that determines the unique traits possessed by organisms. The resulting sequence includes all regions such as exons, introns, regulator, and promoter, which often leads to a vastly large amount of genome information [ 5 ]. With the emergence of next-generation sequencing (NGS) and some other omics technologies used to examine plants genomics, more and more sequenced plants genome will be revealed [ 1 , 6 – 8 ]. To deal with these vast amounts of data, the development and implementation of bioinformatics allow scientists to capture, store, and organize them in a systematic database [ 1 , 5 ].
Bioinformatics databases and tools for plant biotechnology
In the field of bioinformatics, there are a variety of options of databases and tools that are available to perform analysis related to plant biotechnology. Next-generation sequencing (NGS) and bioinformatics analysis on the plant genomes over the years have generated a large amount of data. All these data are submitted to various and multiple databases that are publicly available online. Each database is unique and has its focus. For instance, CottonGen, database is solely dedicated to obtaining genomics and breeding information of any cotton species of interest [ 9 ]. The establishment of such database eases the researchers who are working on cotton genomic studies by focussing on using just one database instead of searching through other available databases. However, some databases are established and designed to cater not only to one specific species or genus, but focus on all the plant species, such as the National Center for Biotechnology Information (NCBI) ( https://www.ncbi.nlm.nih.gov/ ) database, which as of 2021 possesses almost 21,000 plant genomes that are available for access [ 10 ]. Such a database is useful for studies that do not focus on one specific genus or species. This eases the researchers in accessing to all kinds of genomic data in one database. This section will briefly discuss some of the available plant genome databases, which are publicly accessible and not designated for one genus or species alone.
First would be the globally known and recognized database by all the researchers and biologists, which is the NCBI database. NCBI has been dedicated for gathering and analysing information about molecular biology, biochemistry, and genetics. In the NCBI database, one can download the genome information of the plant species of interest from either gene expression omnibus (GEO) ( https://www.ncbi.nlm.nih.gov/geo/ ) or sequence read archive (SRA) ( https://www.ncbi.nlm.nih.gov/sra ) by simply stating the scientific name of the plant in the search bar and the entire genomic information of the plant can then be obtained. The GEO and SRA comprise processed or raw gene expression data or RNA sequencing of plants that are reposited in the repository. For instance, to obtain the genomics of Rosa chinensis (Rose plant), by inputting the name in the search bar, it will direct to the search result page where the researcher can select the most recent or suitable datasets with specific accession number. Depending on the profiling platform used in each dataset, researchers could retrieve either gene symbols, Ensemble ID, open reading frame, chromosomal location, regulatory elements, etc. The information allows researcher to further analyse the subject of study using bioinformatics tools such as gene ontology ( http://geneontology.org/ ), Database for Annotation, Visualization and integration Discovery (DAVID) ( https://david.ncifcrf.gov/ ), Basic Local Alignment Search Tool (BLAST) ( https://blast.ncbi.nlm.nih.gov/Blast.cgi ), and others that is relevant for the study.
Another database that is available for accessing plant genome database is EnsemblPlants ( https://plants.ensembl.org/index.html ). Unlike the NCBI database, which is not only dedicated to plant genomes, EnsemblPlants is specifically dedicated to accessing plant genomes. EnsemblPlant is part of the Ensembl project that started in 1999, where the project aimed to automatically annotate the genome and integrate the outcome of the annotation with other publicly available biological data and establish an open access archive or database online for the use of the research community [ 11 ]. Ensembl project later launched the taxonomic specific websites designated for each taxon under their project that also includes the plants. The database is a user-friendly integrative platform, where it is continuously updated with the new addition of plant species every time a plant genome is completely sequenced. Compared to the NCBI database mentioned earlier, EnsemblPlant not only provides genome sequence, gene models, and functional annotation of the plant species of interest, but also includes the polymorphic loci, population structure, genotype, linkage, and phenotype information [ 11 , 12 ]. Unlike, NCBI, EnsemblPlant does also provide comparative genomics data of the plant species of interest. This indicates that the platform does not only offer genome sequence data but provide additional analytical data about the plant species of interest and help the researchers who are working on plant bioinformatics to save a lot of time by reducing the tedious work in running the analysis. Yet, the researchers could re-assess the data if necessary, depending on the stringency of their work.
Aside from the abovementioned databases that are widely used for retrieving plant genome sequence, there are still other plant databases such as PlantGDB, MaizeDIG, and Phytozome that can also be considered. Table Table1 1 lists the available database and tools that are widely applied in plant biotechnology.
List of bioinformatics databases and tools applied in plant biotechnology
Function | Servers | URL | Details |
---|---|---|---|
Genome Database | ArrayExpress | Archive for functional genomics data from microarray and sequencing platforms | |
BarleyGenes | Gene and RNA-seq database for Barley | ||
Chrysanthemum Transcriptome Database | Database of chrysanthemum’s gene and transcripts | ||
Cottongen | Genomics, genetics, and breeding database for cotton | ||
Expression Atlas EMBL-EBI | Open resource platform for gene and protein expression information | ||
Ensembl Plants | Genomic database (genome sequence, gene models, functional annotation) of more than 33 plant species | ||
Gene Expression Omnibus | Functional genomics data repository | ||
MaizeDIG | Genotypic-phenotypic database for maize | ||
MaizeGDB | Genetic and genomics database for maize | ||
MaizeMine | Archive to access literature, genomic, interaction and proteomic data for maize | ||
VvGDB | Database for grape genome | ||
Phytozome | Comparative genomics portal of plants | ||
Plant Promoter Database | Database of plant promoter sequences and experimentally determined transcription start site of various plant species | ||
PLEXdb | Plant expression database with gene expression profile data sets, structural genomics, and phenotypic data | ||
Pomamo | Archive of potato sequences, literature, maps, and tools | ||
PRGdb | Archive of pathogen receptor genes of various plant species | ||
Rice Expression Database | Repository of gene expression profiles of rice from RNA-seq data on tissues spanning an entire range of rice growth | ||
Rice expression profile Database | Repository of gene expression profiles of rice from microarray analysis | ||
SolGenomics Network | Database and tool of genomics and genetics approach for tomato and some other plant species such as eggplant | ||
TAIR | Database of genetic and molecular biology data for | ||
The Rice Annotation Project Database | Archive of rice genome sequence, structure, and function | ||
Tomato Functional Genomics Database | Archive of tomato microarray data, metabolite and RNA-seq | ||
Metabolomic Database | Metabolights | Metabolomics database (cross-species, metabolite structures, biological role) | |
Pathway Database | Reactome | Pathway interaction repository | |
RiceCyc | Database of known and predicted biochemical pathways from rice | ||
PlantCyc | Metabolic pathway reference database from over 350 plant species | ||
RNA Analysis Tool | qTeller | Comparative RNA-seq expression tool | |
Chemical Compound Database | ChEBI | Archive of molecular entities that focuses on small chemical compounds | |
PubChem | Archive of chemical information of various chemical compounds | ||
Mass Spectrum Database | ReSpect for Phytochemicals | Database for phytochemicals MS spectra data and literature | |
Metlin | Comprehensive MS/MS database | ||
Resistance Analysis | Disease Resistance Analysis and Gene Orthology (DRAGO2) | Annotate resistance genes | |
Networking and Interaction Analysis | PathoPlant | Database of plant-pathogen interaction and components related to plant pathogenesis | |
AraNet | Probabilistic functional gene network tool for | ||
PLANET | Platform for visualization and analysis of co-function networks of photosynthetic organisms | ||
Genomics Tool | The Bio-Analytic Resource | Plant bioinformatics tools resource platform (gene expression, mapping, molecular markers, and genomics) |
Biotechnology and bioinformatics for plant breeding
Plant breeding can be defined as the changing or improvement of desired traits in plants to produce improved new crop cultivars for the benefits of humankind [ 8 ]. Jhansi and Usha [ 13 ] mentioned a few benefits brought by genetically engineered plants such as improved quality, enhanced nutritional value, and maximized yield. The revolution of life science in molecular biology and genomics has enabled the leaps forward in plant breeding by applying the knowledge and biological data obtained in genomics research on crops [ 6 , 8 , 13 ]. In modern agriculture, transgenic technology on plants refers to genetic modification, which is done on plants or crops by altering or introducing foreign genes into the plant, to make them useful and productive and enhance their characteristic [ 13 , 14 ]. As mentioned above, the evolution of next-generation sequencing (NGS) and other sequencing technologies produces a large size of biological data which require databases to store the information. The accessibility of whole genome sequences in databases allows free association across genomes with respect to gene sequence, putative function, or genetic map position. With the aid of software, it is possible to formulate predictive hypothesis and incorporate the desired phenotypes from a complex combination into plants by looking at those genetic markers which score well and gives a higher reliability in breeding [ 2 , 15 ]. Other than genome sequence information, databases which store the information of metabolites also play a crucial role in the study of interaction with proteomics and genomics to reflect the changes in phenotype and specific function of an organism [ 1 ]. Some of the most widely used metabolomics databases for plants and crops such as Metlin ( http://metlin.scripps.edu ), provides multiple metabolite searching and about 240,000 metabolites, nearly 72,000 high-resolution MS/MS spectra, and PlantCyc ( https://plantcyc.org/ ), a database which stores information about biochemical pathway and their catalytic enzyme and genes from plants [ 1 , 16 ]. Moreover, single-nucleotide polymorphism markers also benefit from the revolution of NGS and other sequencing technologies. By using NGS, RNA sequencing (RNA-seq) allows direct measure of mRNA profile in order to identify known single-nucleotide polymorphism (SNP) [ 1 ]. SNP is the unique allelic variation within a genome of same species, which can be used as biological markers to locate the genes associated with desired traits in plants [ 17 , 18 ]. Besides, transcriptome resequencing using NGS allows rapid and inexpensive SNP discovery within a large, complex gene with highly repetitive regions of a genome such as wheat, maize, sugarcane, avocado, and black currant [ 17 ]. Figure Figure1 1 illustrates briefly the process involved in plant breeding using NGS and bioinformatics.

Brief process of plant breeding involving NGS and bioinformatics
Ever since the first transgenic rice production in 2000, there has been a significant revolution in crop genome sequencing projects, along with the advancement in technologies, rapidly increasing the pace in genetically modified organism (GMO) [ 2 , 13 , 19 ]. Among all the products in rice biotechnology, one of the most widely known GM rice is golden rice. Golden rice is a variety of rice engineered by introducing the biosynthetic pathway to produce β-carotene (pro-vitamin A) into staple food in order to resolve vitamin A deficiency. The World Health Organization has classified vitamin A deficiency as public health problem as it causes half a million of children to childhood blindness [ 13 ]. Vitamin A is an essential nutrient to humans as it helps with development of vision, growth, cellular differentiation, and proliferation of immune system; insufficient intake of vitamin A may lead to childhood blindness, anaemia, and reduced immune responsiveness against infection [ 20 ]. Being the first crop genome to be sequenced, rice has become the most suitable model to initiate the development and improvement of other species in genomic aspect [ 21 – 24 ]. The particular reason is due to its small genome size and diploidy, which enables rice to be an excellent model for other cereal crops with larger genomes, such as maize and wheat [ 21 , 23 ]. Song et al. [ 22 ] reported the complete genome sequence of two rice subspecies, japonica and indica , in 2005 that laid a strong foundation for molecular studies and plant breeding research [ 22 , 24 ]. With recent advancement in bioinformatics, it is now possible to run the sequence alignment between large and complex genome from other crop species with genomic data available from rice, by using different software or tools, in order to find out the shared conserved sequence through comparative genomics [ 2 , 7 ]. Vassilev et al. stated some of the most commonly used programmes such as BLAST and FASTA format allowed rapid sequence searching in databases and give the best possible alignment to each sequence [ 25 ]. The programming algorithm calculates the alignment score to measure the proportion of homology matching residue between sequence from related species [ 2 ].
Wheat, as the most widely grown consumed crops, together with rice and maize contributes more than 60% of the calories and protein for our daily life [ 26 , 27 ]. To meet the demands of human population growth, it is necessary to achieve more understanding in wheat research and breeding in order to accelerate the production of wheat yield by 2050 [ 26 – 28 ]. Despite its importance, the improvement of wheat has been challenging as the researchers have to overcome the complexity of the wheat genome such as highly repetitive and large polyploid in order to get a fully sequenced reference genome [ 26 , 29 ]. Advances in next-generation sequencing (NGS) platforms and other bioinformatics tools have revealed the extensive structural rearrangements and complex gene content in wheat, which revolutionized wheat genomics with the improvement of wheat yield and its adaptation to diversed environments [ 26 , 29 ]. The NGS platforms allow the swift detection of DNA markers from the huge genome data in a short period of time. These NGS-based approaches have undoubtedly revolutionized the allele discovery and genotype-by-sequencing (GBS). By providing a high-quality reference genome of wheat in databases, it allows more sequence comparison between wheat and other species to find out more homologous gene. Moreover, the development of sequencing technologies in both high-throughput genotyping and read length, combining with biological databases, allow the rapid development of novel algorithm to complex wheat genome [ 29 , 30 ]. For instance, genome-wide association studies (GWAS) are an approach used in genome research which allows rapid screening of raw data to select specific regions with agronomic traits [ 29 , 31 ]. It allows multiple genetic variants across genome to be tested to study the genotype-phenotype association; thus, this method can be used to facilitate improvement in crop breeding via genomic selection and genetic modification [ 16 , 29 ].
Maize, a globally important crop, not only has a wide variety of uses in terms of economic impact, but can also serve as genetic model species in genotype to phenotype relationship in plant genomic studies [ 32 , 33 ]. Besides, due to its extremely high level of gene diversity, maize has high potential in the improvement of yield to meet the demands of population growth [ 33 ]. Despite the combination of economic and genomic impact, the progress in generating a whole genome sequence in maize has been a computational challenge due to the presence of tremendous structural variation (SV) in its genome [ 34 ]. The introduction of NGS techniques in several crops including maize allowed the rapid de novo genome sequencing and production of huge amount genomics and phenomics information [ 1 , 35 ]. A better integration of data within multiple genome assemblies is much needed to study the connection between phenotype and genotype in order to achieve yield and quality improvement of maize [ 35 ]. Nowadays, some user-friendly online databases such as qTeller, MaizeDIG, and MaizeMine are designed to ease the comparison and visualization of relationships between genotypes and phenotypes [ 36 ]. MaizeGDB, a model organism database for maize, provides the access of data on genes, alleles, molecular markers, metabolic pathway information, phenotypic images with description, and more which are useful for maize research [ 35 , 36 ]. MaizeMine is a data mining resource under MaizeGDB, which was designed to accelerate the genomics analysis by allowing the researchers to better script their own research data in downstream analysis [ 36 ] whereas MaizeDIG is a genotype-phenotype database which allows the users to link the association of genotype with phenotype expressed by image [ 35 , 36 ]. Cho et al. [ 35 ] reported that with the accessibility via image search tool, the relationship between a gene and its phenotype features can be visualized within image. The integration and visualization of high-quality data with these tools enables quick prioritizing phenotype of interest in crops, which play a crucial role in the improvement of plant breeding.
Bioinformatics for studying stress resistance in plants
The understanding of the stress response on plants is vital for the improvement of breeding efforts in agriculture, and to predict the fate of natural plants under abiotic change especially in the current era of continuous climate change [ 37 ]. Stress response in plants can be divided into biotic and abiotic. Biotic stress mainly refers to negative influence caused by living organism such as virus, fungi, bacteria, insects, nematodes, and weeds [ 38 ] while abiotic stress refers to factors such as extreme temperature, drought, flood, salinity, and radiation which dramatically affect the crop yield [ 37 ]. NGS technologies and other potent computational tools, which allowed sequencing of whole genome and transcriptome, have led to the extensive studies of plants towards stress response on a molecular basis [ 1 , 2 , 37 ]. The tremendous amount of plant genome data obtained from genome sequencing allows the investigation of correlations between the molecular backbone of living organism and their adaptations towards the environment [ 16 ].
Biotic and abiotic stress management
How the plants and crops respond towards stress environment is the key to ensure their growth and development, and to avoid the great crop yield penalty caused by harsh condition [ 35 , 39 ]. Therefore, the utilization of bioinformatic tools is important to study and analyse the plant transcriptome in response to biotic and abiotic stress. Besides, the application of bioinformatics tools on plants and crops genome can benefit the agricultural community by searching the desired gene among genome from different species and elucidate their function on the crops [ 35 ]. The genome databases play a crucial role in storing and mining large and complex genome sequence from the plants. Besides data storage, some genome databases are also able to perform gene expression profiling to predict the pattern of gene expressed at the level of transcript in cell or tissues. By using in silico genomic technologies, the disease resistance gene-enzyme with their respective transcription factor, which plays a role in defence mechanism against stress, are able to be identified [ 40 , 41 ]. For instance, a large-scale transcriptome sequencing of chrysanthemum plants was carried out by Xu et al. [ 40 ] to study the dehydration stress in chrysanthemum plants. An online database called Chrysanthemum Transcriptome Database ( http://www.icugi.org/chrysanthemum ) was developed to allow the storage and distribution of transcriptome sequence and its analysis result among research community [ 40 ]. With the aid of different protein databases, the biochemical pathway and kinase activity of chrysanthemum in response to dehydration stress are able to be predicted [ 40 ]. Xu et al. [ 40 ] also reported a total of 306 transcription factor and 228 protein kinase that are important upstream regulator in plants when encountered with various biotic and abiotic stresses.
Bioinformatics approaches to study resistance to plant pathogen
One of the challenges in modern agriculture to supply the nutrition’s demand along with the world population growth is the crop loss due to disease. The study of plant pathogen plays an essential role in the study of plant diseases, including pathogen identification, disease aetiology, disease resistance, and economic impact, among others [ 41 ]. Plants protect themselves through a complex defence system against variety of pathogen, including insects, bacteria, fungi, and viruses. Plant-pathogen interaction is a multicomponent system mediated by the detection of pathogen-derived molecules in the form of protein, sugar, and polysaccharide, by pattern recognition receptor (PRRs) within the plants [ 42 – 45 ]. After the recognition of enemy molecules, signal transduction is carried out accordingly and plant immune systems will respond defensively through different pathways involving different genes [ 42 ]. According to Schneider et al. [ 46 ], the development of molecular plant pathology can be broadly divided into three eras, begins with the disease physiology starting from early 1900s until 1980s [ 46 ]. In the second era of molecular plant genetic studies, one or a few genes of bacterial pathogens were focused whereas the third era of plant genomic studies began in 2000 with the sequencing of genome, and the first complete genome of bacterial pathogen, Xylella fastidiosa , was obtained [ 46 ]. The recent advance in DNA sequence technologies allow researchers to study the immune system of plants on genomic and transcriptomics level [ 1 , 41 , 42 ]. Genomics has revealed the mystery and complexity and consequently the various information about phytopathogen. A clearer picture of plant-pathogen interactions in the context of transcriptomic and proteomics can be visualized through the application of different bioinformatics tools, which in turn made feasible the engineering resistance to microbial pathogen in plant [ 43 ].
PRGdb: bioinformatics web for plant pathogen resistance gene analysis
Plants have developed a wide range of defence mechanism against different pathogen and ultimately inhibit growth and spread of pathogen [ 47 , 48 ]. Plant defence system is mediated by resistance (R) gene [ 47 ]. R gene plays an important role in defence mechanism. They encode for protein that recognizes specific avirulent (Avr) pathogen proteins and initiated the defence mechanism through one or more signal transduction pathway in a hypersensitive response (HR) [ 41 , 47 , 48 ]. However, the essential components needed for protein to exert their resistance are still unidentified [ 48 ]. With the intention to study and identify more novel R gene, high-throughput genomic experiments and plant genomic sequence are essential to explore their function and new R gene discovery [ 47 ]. In 2009, Plant Disease Resistance Gene database (PRGdb), a comprehensive bioinformatics resource across hundreds of plant species, was launched in order to facilitate the plant genome research on discovery and predict plant disease resistance gene [ 47 , 48 ]. To date, PRGdb 3.0 has been released with 153 reference resistance genes and 177,072 annotated candidate pathogen receptor genes (PRGs) [ 49 ]. This database act as an important reference site and repository to all the research studies on exploration and use of plant resistance genes [ 48 , 49 ].
Apart from resistance gene storage, this easily accessible platform also allows different tools that are essential for exploration and discovery of novel R gene. For instance, the DRAGO 2.0 tool, which was built to explore known and novel disease resistance gene, can be launched on any transcriptome or proteome to annotate and predict PRG from DNA or amino acid with high accuracy [ 49 ]. Besides, BLAST search tools available in PRGdb provide comparison of different sequences which allowed the determination of gene homology and expression analysis. Apart from the database, plant pathology field also benefited from whole genome sequence technologies. The new DNA sequencing technologies such as NGS and Sanger sequencing allowed the study of genomics, proteomics, metabolomics, and transcriptomics on both the host plant and the pathogen [ 1 ]. The phytopathogen genomes which have been sequenced are expected to provide valuable information on the molecular basis for infection of plant host and explore the potential novel virulence factors [ 1 ]. Figure Figure2 2 illustrates a brief process involved in producing stress-resistant plant using bioinformatics approach.

Brief process involved in producing stress-resistant plant using bioinformatics approach
Metagenomics in plant biotechnology and Cas9 modification
The effects of environment microorganisms’ community, especially soil microorganism on plants, may contribute to plant’s growth and pathogenesis. Through metagenomics approaches, the soil microorganism community that contributed to plant growth may provide a great genomic insight into physiology and pathology [ 50 – 53 ]. In metagenomics approaches, the overall genetic materials obtained from soil are sequenced and advancing to microbial community analysis via data analytics [ 53 – 55 ]. The extracted genetic materials from the soil were subjected to high-throughput metagenomics analysis via various NGS approaches such as 16S rRNA sequencing, shotgun metagenomic sequencing, MiSeq sequencing [ 54 – 56 ] for microbial species identification, functional genomics study, and structural metagenomic analysis. A NGS produces huge genomics data for each study; thus, application of bioinformatics tools would add value in the metagenomics analysis as the target genes identified could advance into elucidation of plant growth, plant disease, soil contamination, and microbial taxonomy [ 52 ]. For example, the use of UNITE ( https://unite.ut.ee/ ) for fungi identification [ 57 ], SILVA ( https://www.arb-silva.de/ ) for 16S rRNA [ 58 ], and MGnify ( https://www.ebi.ac.uk/metagenomics/ ) possesses metagenomics data of microbiome [ 59 ]. These databases allow the researchers to retrieve and analyse the relevant metagenomic sequenced data for a specific study.
Since metagenomics analysis provides the greater output on plant-microbe interaction, the genes that are responsible for plant immunity may play a crucial role in protecting against disease-causing microorganism [ 60 , 61 ]. With the emergence of Clustered Regularly Interspaced Short Palindrome Repeats (CRISPR) gene editing technique, Cas9 modification could produce a better plant trait and disease-resistant plant [ 62 , 63 ]. The CRISPR/Cas9 system is employed in studying the functional genomics in plants in relation to plant-microbe interaction. CRISPR/Cas9 system facilitated the gene editing by creating a mutant through double-stranded break forming a targeted gene mutation and followed by genome repair [ 63 – 65 ]. The CRISPR/Cas9 modification on OsSWEET14 genes protects the Super Basmati Rice from bacterial blight causes by Xanthomonas oryzae pv. oryzae [ 66 ]. Gene editing to knockout OsMPK5 and OsERF922 genes in rice protects against Magnaporthe grisea and Magnaporthe oryzae , respectively [ 67 – 69 ]. Besides that, Cas9 modification on Cs WRKY22 and TcNPR3 increased host defence immunity through regulating salicylic acid in Citrus sinensis and Theobroma cacao , respectively [ 70 , 71 ]. Thus, CRISPR/Cas9 modification could be one of important science advancements to validate the metagenomics analysis on plant-microbe interaction.
Current challenges of bioinformatics applications in plant biotechnology
Despite the beneficial prospect of the bioinformatics applied in plant biotechnology, there are many challenges and limitations must be addressed in order to fully utilize their potentials [ 1 ]. Along with the rapid growth in plant genome data mining and database development, there are a few challenges faced by bioinformaticians and scientists which can be divided into number of areas as mentioned in the subsections below.
Bioinformatic data management and organization and synchronize update resources
Since the introduction of the next-generation sequencing (NGS), which is commercially available in 2004, enormous amount of data has been generated in plant genome research. Thousands of Gb of plants sequences are deposited in various public databases monthly [ 1 , 72 , 73 ]. Moreover, the constantly sequenced and re-sequenced of the plant genome has developed a vast amount of new genome sequence in all public databases. The increase in sequenced plant genome driven by technological improvement has led to a problem that arises along with the storage and update of a large amount of data [ 72 , 74 ]. The update process should occur in all the comparative databases, not just solely individual genome database [ 72 ]. With this, the synchronized update of genome data resources among different plant genomic platform is able to provide a strong, updated, reliable database community that all the plant researchers can rely on [ 72 ].
Complexity of plant genetic content
Other than the tremendous amount of genome sequence generated, the complexity of the plant genetic content is also a challenging issue faced by plant research community. Even though the arrival of next-generation sequencing technologies has allowed the rapid DNA sequencing for non-model or orphan plant species, the sequencing pace for plants is far from that of animal and microorganism [ 74 ]. The main factor which contributes to this situation is because sometimes the plant genome can be nearly hundred times larger than the currently sequenced animal and microorganism genome [ 73 ]. Needless to say, some of the plant genome even can have polyploidy, a duplication of an entire genome, which is estimated to occur in 80% of the plant species [ 73 , 75 ]. According to Schatz et al., the genome assembly in the case of large size plant genome with abundance of repetitive sequence can be metaphorically described as build-up of a large puzzle consisting of blue sky separated by nearly indistinguishable white clouds of small gene [ 73 ]. The particular reason for this is mainly because the sequence length in NGS is relatively shorter than in Sanger sequencing and required dedicated assembly algorithm [ 74 ]. Therefore, most plant genomes sequenced by NGS can only be used for establishing gene catalogues, interpreting the repeat content, glimpsing evolutionary mechanism, and performing on comparative genomics in early study [ 74 ].
Advance in sequencing technologies
There are two basic approaches to genome assembly, i.e. comparative genome assembly and de novo genome assembly [ 75 ]. It is important to distinguish between these two different approaches. Comparative is a reference-guided method which use a genome or transcriptome, or both, for guidance, whereas de novo assembly refers to reconstruction of a genome from organisms that have not been sequenced before [ 74 , 75 ]. Table Table2 2 compares some of the available assembly and NGS technology available for genome sequencing. However, these two approaches are not completely exclusive due to a lack of bioinformatic tools designed to cope with the unique and challenging features of plant genomes [ 74 , 75 ]. One of the biggest challenges in the development of bioinformatic software is the algorithm development [ 76 ]. As is known, all the programmes or software in bioinformatic are very computationally intensive. As most of the assemblies available now solely rely on single assembly, a development in better algorithm in terms of resource requirement is essential for combining different assemblers by using a different underlying algorithm in order to give a more credible final assembly [ 74 , 76 ].
Comparison between next-generation sequencing technologies
Method | Illumina | Pacific Bio | Nanopore | Pyrosequencing (454) | SOLiD |
---|---|---|---|---|---|
Read length per run | 50–300 base pair | 10–25 kilo base pair | 500–2.3 mega base pair | Approximate 800 base pair | 50 base pair |
Time taken per run | 1 to 10 days | Up to 30 h | 1 min–72 h | 24 h | 1 to 2 weeks |
Cost | $148 per Gb | $2000 Gb | $60–80 per sample | $7000 per sample | $15,000 per 100 Gb |
Accuracy | 98% | 99.9% | 98.9–99.6% | 99.9% | 99.9% |
Advantages | Cost-effective, high-yield sequence reads | Fast, long read lengths | Real-time analysis, long read lengths | Fast, long read lengths | High accuracy |
Disadvantages | Instrument cost, high maintenance of instrument, read length | Low high throughput | Error prone | Homopolymer error | Long run time, low read length |
Database accessibility
To date, there are about 374,000 known plant species in the world [ 77 ]. The first full plant genome sequencing was completed on A rabidopsis thaliana through Sanger sequencing methods in 2000 [ 78 ]. Although introduction of molecular biology decades ago may have facilitated the species identification, obtaining the full plant genomic data remains challenging due to the genome complexity. The development of NGS platform may foster the plant genome sequencing, yet there are limited sequenced datasets reposited to the database. To date, there are only 29 plant genome databases accessible in PlantGDB genome browser allowing researchers to retrieve the information about gene structure, matched GSS contigs, similar protein, spliced alignments EST, etc. Besides, the PlaD database ( http://systbio.cau.edu.cn/plad/index.php ) that focuses on the microarray data of the plants developed by China Agricultural University comprises transcriptomic database for plant defence against pathogen. However, it is limited to Arabidopsis , rice, maize, and wheat [ 79 ]. The Plant Omics Data Center ( http://plantomics.mind.meiji.ac.jp/podc/ ) is another publicly available web-based plant database featuring omics data for co-expressed profile, regulatory network, and plant ontology information [ 80 ]. Although curated omics datasets could be retrieved from PODC, information are restricted for certain plants and crops such as Arabidopsis , tobacco, earthmoss, barrelclover, soybean, potato, rice, tomato, grape, maize, and sorghum. Furthermore, all these publicly available databases require constant updating with new released data or resequencing data so that the researcher could obtain the most updated version of genome datasets for their research.
The application of bioinformatics in plant biotechnology represents a fundamental shift in the way scientists study living organisms. Bioinformatics play a significant role in the development of agriculture sector as it helps to study the stress resistance and plant pathogen, which are critical in advancing crop breeding [ 75 ]. NGS and other sequencing technologies will make more plant genome data accessible in all public databases and enable the identification of genomic variants and prediction of protein structure and function [ 75 , 76 ]. Moreover, GWAS, which allows the identification of loci and allelic variation related to valuable traits, eased the crop modification and improvement [ 74 ]. In brief, the advance in bioinformatics application in plant biotechnology enables researchers to achieve fundamental and systematic understanding of economically important plant. However, despite all these exciting achievement by the application of bioinformatic on plant biotechnology, it is still a long way from automated full genome sequencing and assembly at a low cost [ 76 ]. There is a critical need for effective bioinformatic tools which are able to provide longer reads with unbiased coverage in order to overcome the complexity of the plant’s genome. To achieve this, an enhanced algorithm development is essential to enable data mining and analysis, comparison, and so on. Therefore, bioinformaticians and experts with mathematical and programming skills will play an important role in bringing fresh approaches and knowledge into bioinformatics, not only for the advancement in plant biotechnology and agriculture sector, but the future of humanity as well.
Acknowledgements
The authors wish to thank Prof. Hoe I. Ling of Columbia University (New York, USA) for his editorial input and proofread the manuscript.
Abbreviations
GWAS | Genome-wide association studies |
NGS | Next-generation sequencing |
PRGdb | Plant Disease Resistance Gene database |
RNA-seq | RNA sequencing |
SNP | Single-nucleotide polymorphism |
Authors’ contributions
YCT designed the content and was a major contributor in writing the manuscript. AUK and YPW edited the manuscript. APKL designed and edited the manuscript. All authors read and approved the final manuscript.
Availability of data and materials
Declarations.
The authors declare that they have no competing interests.
Publisher’s Note
Springer Nature remains neutral with regard to jurisdictional claims in published maps and institutional affiliations.
Recent Advances in Plant Biotechnology
- © 2009
- Ara Kirakosyan 0 ,
- Peter B. Kaufman 1
University of Michigan, Ann Arbor, U.S.A.
You can also search for this author in PubMed Google Scholar
- Presents a full overview of plant biotechnology from the history to applications
- Approach includes associated risks and the effects of plant biotechnology on global warming, alternative energy initiatives, food production, and medicine
- Includes supplementary material: sn.pub/extras
33k Accesses
81 Citations
4 Altmetric
This is a preview of subscription content, log in via an institution to check access.
Access this book
Subscribe and save.
- Get 10 units per month
- Download Article/Chapter or eBook
- 1 Unit = 1 Article or 1 Chapter
- Cancel anytime
- Available as EPUB and PDF
- Read on any device
- Instant download
- Own it forever
- Compact, lightweight edition
- Dispatched in 3 to 5 business days
- Free shipping worldwide - see info
- Durable hardcover edition
Tax calculation will be finalised at checkout
Other ways to access
Licence this eBook for your library
Institutional subscriptions
About this book
Similar content being viewed by others.
Plant Genetics and Molecular Biology: An Introduction
History of Plant Biotechnology Development
- agriculture
- alternative energy
- bioremediation
- biotechnology
- genetically modified plants
- herbal medicine
- herbal products
- plant biotechnology
- transgenic plants
Table of contents (16 chapters)
Front matter, plant biotechnology from inception to the present, overview of plant biotechnology from its early roots to the present.
- Ara Kirakosyan, Peter B. Kaufman, Leland J. Cseke
The Use of Plant Cell Biotechnology for the Production of Phytochemicals
- Ara Kirakosyan, Leland J. Cseke, Peter B. Kaufman
Molecular Farming of Antibodies in Plants
- Rainer Fischer, Stefan Schillberg, Richard M. Twyman
Use of Cyanobacterial Proteins to Engineer New Crops
- Matias D. Zurbriggen, Néstor Carrillo, Mohammad-Reza Hajirezaei
Molecular Biology of Secondary Metabolism: Case Study for Glycyrrhiza Plants
- Hiroaki Hayashi
Applications of Plant Biotechnology in Agriculture and Industry
New developments in agricultural and industrial plant biotechnology, phytoremediation: the wave of the future.
- Jerry S. Succuro, Steven S. McDonald, Casey R. Lu
Biotechnology of the Rhizosphere
- Beatriz Ramos Solano, Jorge Barriuso Maicas, Javier Gutierrez Mañero
Plants as Sources of Energy
- Leland J. Cseke, Gopi K. Podila, Ara Kirakosyan, Peter B. Kaufman
Use of Plant Secondary Metabolites in Medicine and Nutrition
Interactions of bioactive plant metabolites: synergism, antagonism, and additivity.
- John Boik, Ara Kirakosyan, Peter B. Kaufman, E. Mitchell Seymour, Kevin Spelman
The Use of Selected Medicinal Herbs for Chemoprevention and Treatment of Cancer, Parkinson’s Disease, Heart Disease, and Depression
- Maureen McKenzie, Carl Li, Peter B. Kaufman, E. Mitchell Seymour, Ara Kirakosyan
Regulating Phytonutrient Levels in Plants – Toward Modification of Plant Metabolism for Human Health
Risks and benefits associated with plant biotechnology, risks and benefits associated with genetically modified (gm) plants.
- Peter B. Kaufman, Soo Chul Chang, Ara Kirakosyan
Risks Involved in the Use of Herbal Products
- Peter B. Kaufman, Maureen McKenzie, Ara Kirakosyan

Risks Associated with Overcollection of Medicinal Plants in Natural Habitats
- Maureen McKenzie, Ara Kirakosyan, Peter B. Kaufman
Authors and Affiliations
Ara Kirakosyan, Peter B. Kaufman
About the authors
Bibliographic information.
Book Title : Recent Advances in Plant Biotechnology
Authors : Ara Kirakosyan, Peter B. Kaufman
DOI : https://doi.org/10.1007/978-1-4419-0194-1
Publisher : Springer New York, NY
eBook Packages : Biomedical and Life Sciences , Biomedical and Life Sciences (R0)
Copyright Information : The Editor(s) (if applicable) and The Author(s), under exclusive license to Springer Science+Business Media, LLC, part of Springer Nature 2009
Hardcover ISBN : 978-1-4419-0193-4 Published: 30 July 2009
Softcover ISBN : 978-1-4899-7916-2 Published: 23 August 2016
eBook ISBN : 978-1-4419-0194-1 Published: 15 August 2009
Edition Number : 1
Number of Pages : XIV, 405
Topics : Plant Genetics and Genomics , Plant Sciences
- Publish with us
Policies and ethics
- Find a journal
- Track your research

- Journal home
- Advance online publication
- About the journal
- J-STAGE home
- Plant Biotechnology

Near-complete genome assembly of tomato ( Solanum lycopersicum ) cultivar Micro-Tom
Released on J-STAGE: July 19, 2024 | Article ID 24.0522a
Kenta Shirasawa, Tohru Ariizumi
Creative Ecology
Released on J-STAGE: April 30, 2010 | Volume 16 Issue 1 Pages 15-25
Akira Miyawaki

TALE-based C-to-T base editor for multiple homologous genes with flexible precision
Released on J-STAGE: July 02, 2024 | Article ID 24.0510a
Ayako Hosoda, Issei Nakazato, Miki Okuno, Takehiko Itoh, Hideki Takanashi, Nobuhiro Tsutsumi, Shin-ichi Arimura
Gateway vectors for plant transformation
Released on J-STAGE: June 27, 2009 | Volume 26 Issue 3 Pages 275-284
Tsuyoshi Nakagawa, Sumie Ishiguro, Tetsuya Kimura
The floral inoculating protocol: a simplified Arabidopsis thaliana transformation method modified from floral dipping
Released on J-STAGE: September 25, 2010 | Volume 27 Issue 4 Pages 349-351
Mari Narusaka, Tomonori Shiraishi, Masaki Iwabuchi, Yoshihiro Narusaka
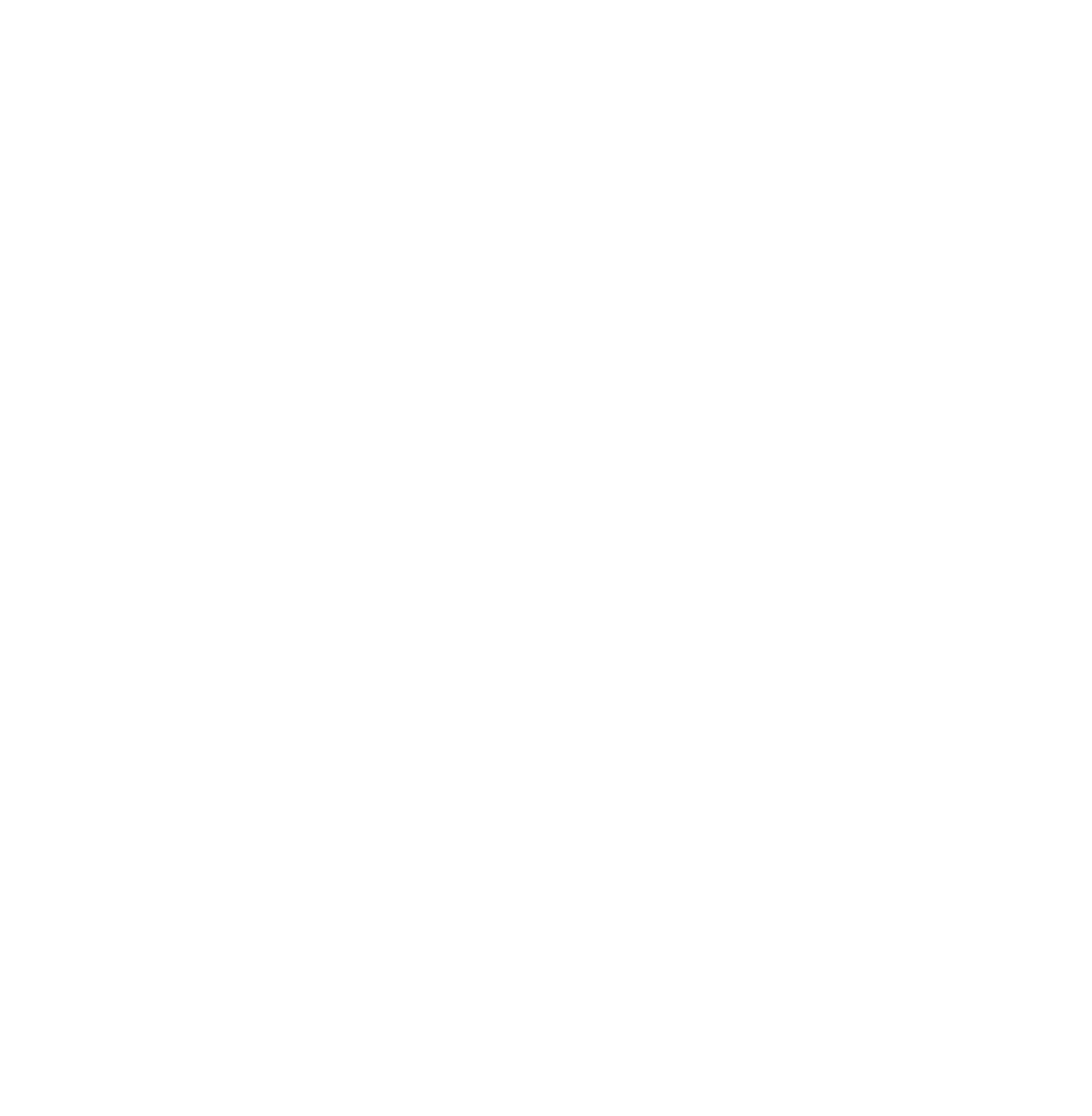
- Volume Vol 41 Vol 40 Vol 39 Vol 38 Vol 37 Vol 36 Vol 35 Vol 34 Vol 33 Vol 32 Vol 31 Vol 30 Vol 29 Vol 28 Vol 27 Vol 26 Vol 25 Vol 24 Vol 23 Vol 22 Vol 21 Vol 20 Vol 19 Vol 18 Vol 17 Vol 16 Vol 15 Vol 14
- Add to favorites
- Announcement alert
- New arrival alert
Plant tissue culture letters
Register with J-STAGE for free!
Already have an account? Sign in here
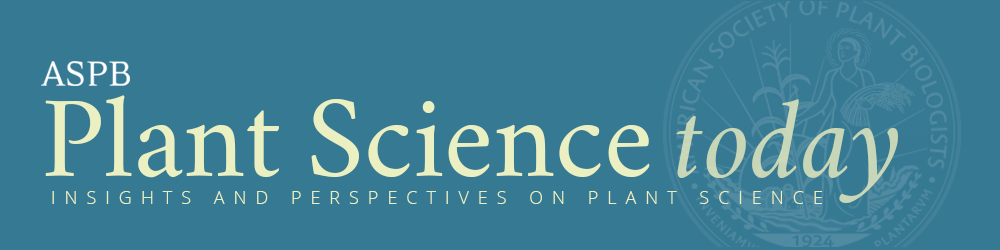
25 Inspiring Women in Plant Biology
In March, the ASPB Women in Plant Biology Committee asked you to nominate remarkable women who shape our field for recognition by the ASPB community. We recognize these 25 women to highlight their achievements and leadership, celebrate their impact in plant biology, and inspire future generations. We will continue to build a more equitable and inclusive plant biology community.
Distinguished Researchers
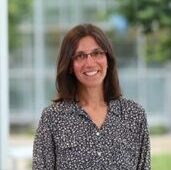
Anjali Iyer-Pascuzzi Biotic Interactions: Plant-Microbe Pathogenesis
Dr. Anjali Iyer-Pascuzzi, leading an all-female lab at Purdue University, has achieved significant milestones in her academic career. She currently oversees the research endeavors of six PhD students focusing on plant pathology. Anjali’s research has been diverse and impactful, ranging from investigating the effects of spaceflight and simulated microgravity on plant defense responses in collaboration with NASA to unraveling the infection strategies of various plant pathogens, including Phyllachora maydis and Ralstonia solanacearum. Her work has been recognized and cited extensively, reflecting her profound contributions to the fields of plant biology and microbiology.
“Dr. Iyer-Pascuzzi is a great mentor and creates a supportive lab environment.”
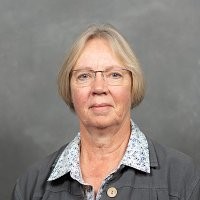
Anne Fennell Abiotic: Light & Temperature
Dr. Anne Fennell’s research on grapevine bud dormancy, cold hardiness, and chilling fulfillment has had significant impacts on the global grapevine research community. Her research focuses on Vitis riparia Michx., a north American species used in grapevine root stocks around the world. Anne has developed a large and diverse populations of intergenic crosses involving this species enabling genetic studies for various complex traits significant for grapevine production under stress. In addition, her group has developed genomic resources including a complete genome for a northern ecotype of this Native American species, that is used in breeding for cold hardiness. Her genuine care for the students and her willingness to go the extra mile in making sure students learn the latest and most practical information necessary are unique qualities that are highly appreciated by students. Anne strives to meet individuals where they are and open and enhance their interest in the world of plant science research. She maintains a diverse lab experience for undergraduates through postdocs with studies in physiology, genetics, genomics, and big data and emphasizes national and international collaborations. This vertical integration of lab members provides undergraduates an opportunity to see opportunities beyond an hourly job as team members move on to careers in higher education and industry. National collaborative grants provide all team members with training in team science in addition to their specific areas of research. Her research program in the perennial grapevine is grounded in basic plant biology and moves seamlessly from the grapevine DNA and RNA back to the field performance/phenotype to identify genotypes, genes, pathways, and genetic architecture which impact sustainable production of the grapevine. Recent research impacts include using an F2 population to identify molecular markers for root architecture and berry enological characters (malic acid, yeast assimilable nitrogen and grassy notes).
“Anne is an exemplary scientist and mentor who strives to collectively advance both the science and the people who do it.” “Anne’s genuine care for her students and her willingness to go the extra mile in making sure students learn the latest and most practical information necessary is highly appreciated.”
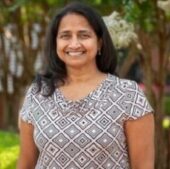
Aruna Kilaru Biochemistry & Metabolism
Dr. Aruna Kilaru’s career is marked by significant contributions to both research and mentorship, shaping the professional development of many. Dr. Aruna Kilaru’s career is distinguished by her groundbreaking research aimed at enhancing crop productivity through the modification of lipid metabolism for storage oil biosynthesis and stress responses. Her work delves into the intricate mechanisms underlying lipid synthesis and signaling, spanning biochemical, cellular, physiological, and molecular levels. Dr. Kilaru’s primary focus lies in elucidating the regulation of storage oil content and composition, alongside investigating lipid-mediated stress response pathways in plants. By leveraging a diverse array of cutting-edge tools, from scanning electron microscopy to “omic” approaches, she endeavors to unravel the complexities of plant lipid metabolism. Through her innovative research endeavors, Dr. Kilaru aims to provide crucial insights into developing stress-tolerant crops with improved oil content and overall yield, thus addressing pressing agricultural challenges. Dr. Kilaru’s impact extends far beyond her scientific endeavors. Her dedication to nurturing the growth of her students and her service to the American Society of Plant Biologists (ASPB) are commendable. Dr. Kilaru’s journey from a village in India to the United States serves as an inspiring example for women globally, showcasing the power of passion and determination in achieving success.
“Dr. Aruna Kilaru has made an indispensable contribution to my professional development. I extend my sincerest gratitude to Dr. Kilaru for her guidance and support.” “A great leader is like the sun, lights your days but enlightens you through the darkest of nights.”
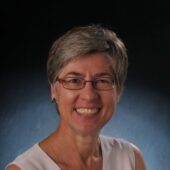
Barbara Demmig-Adams Biochemistry: Photosynthesis & BioEnergy
Dr. Barbara Demmig-Adams is Professor of Distinction in the Department of Ecology & Evolutionary Biology at the University of Colorado at Boulder, USA. She is a David & Lucille Packard Fellow and member of Leopoldina (National Academy of Sciences of Germany, Austria, and Switzerland). Dr. Demmig-Adams is best known for her pioneering role in discovering that the carotenoids of the xanthophyll cycle serve in photoprotection of plants against damage from excess absorbed light. She also contributed to uncovering what limits the capacity of photosynthesis and how manipulation of this capacity may enhance photosynthetic organisms’ productivity and tolerance of challenging environments. Her recent work uses insight from plant ecophysiology to co-optimize nutritional quality and sustainability of novel food systems in the context of climate change. Together with her students, she also synthesizes literature on the benefits of plant-based nutrition (and other aspects of lifestyle medicine) for human wellness and health.
“Dr. Demmig-Adams is someone to look up to as a strong woman in STEM … to build my confidence … with continued support, constant kindness, and compassion.”
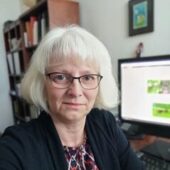
Dorothea Tholl Biochemistry & Metabolism
Dr. Dorothea Tholl is a professor in the department of Biological Sciences at Virginia Tech. She earned her PhD from the Technical University of Braunschweig, Germany, and conducted postdoctoral research at the Max Planck Institute for Chemical Ecology prior to establishing her research program in the US. Her research is focused on the biosynthesis, evolution, and function of specialized metabolites, especially volatile organic compounds, in chemical interactions of plants and animals. She has elucidated key enzymatic steps in the metabolism of plant volatiles with roles in direct and indirect defenses above and belowground. More recently, her team has made investigations in the convergent evolution of volatile metabolism in plants and animals by exploring the biosynthesis of insect pheromones. Applied projects in her lab examine the metabolism of volatile aroma compounds and defense metabolites in root crops and common flavor constituents in spices and culinary herbs. She is also interested in the metabolic engineering of insect pheromones in trap plants or microbial systems to develop novel integrated pest management strategies. Dr. Tholl has enjoyed serving her research community as president of the Phytochemical Society of North America from 2021-2022 and as a member of The Plant Cell editorial board since 2020.
“Dr. Tholl is widely known as an engaged, supportive, and enthusiastic colleague and highly effective mentor for her students and postdocs. She deserves special recognition for her efforts.”
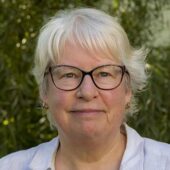
Jane Parker Biotic interactions
Prof. Jane Parker’s career revolves around unraveling the intricate mechanisms of plant innate immunity, aiming to decipher key players and processes that enable plants to effectively combat attacking microbes while maintaining growth and physiological fitness. Jane is based at The Max-Planck Institute for Plant Breeding Research and is Associate Professor at the at the University of Cologne. Sheplays a pivotal role in advancing scientific knowledge and nurturing the next generation of researchers. Her groundbreaking research has revealed the existence of multiple layers of defense, with the EDS1 protein family and its partners identified as crucialdrivers of immune responses after pathogen recognition by host receptors. Jane’s contributions to the field are widely recognized. Additionally, her commitment to scientific excellence is evident through her active involvement in various societies, boards and editorial roles. Jane’s research achievements culminated in her election as a Fellow of The Royal Society, a member of The US Academy of Sciences, and several other esteemed scientific academies and societies.
“I really enjoy the outstanding breakthroughs of Dr. Parker.”
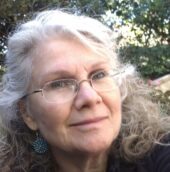
Janet Slovin Genes & Genomes: Genetics
Dr. Janet P. Slovin’s illustrious career includes her pioneering research integrating molecular, biochemical, physiological, and genetic approaches to unravel plants’ fundamental mechanisms. She made significant strides in auxin regulation of plant development, identifying the first plant mutant in IAA levels and contributing to our understanding of the IAA biosynthetic pathway and turnover. Her other research achievements include proving the involvement of low molecular weight heat shock proteins in thermotolerance and, with others pioneering the assembly of the F. vesca genome sequence through one of the first “crowd-sourced” sequencing efforts. Her foresight and collaboration have provided invaluable genomic tools for the Rosaceae research community, cementing her legacy as a visionary in plant science. As a trailblazer for women in plant science, Slovin was instrumental in forming the Women in Plant Physiology/Biology within ASPB and fought for equality within the USDA, winning a significant EEOC case on discrimination. Her contributions extend to organizing the first Mid-Atlantic Plant Molecular Biology Society and participating in outreach activities.
“She was one of the true women pioneers.” “A scientist’s scientist who cares about excellent research and the value women bring to science and society. ”
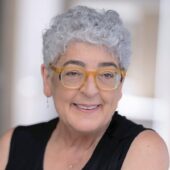
Joanne Chory Signal Transduction & Hormone Biology
Joanne Chory has dedicated over 30 years to studying Arabidopsis thaliana , a small flowering mustard plant, using molecular genetics to explore how plants adapt their size, shape, and form to optimize growth and photosynthesis in various environments. By leveraging genetics, she has been able to enhance two crucial natural characteristics of plants: their root systems and their capacity to produce suberin—a carbon-rich polymer that is naturally resistant to decomposition. By enhancing a plant’s ability to capture and retain more carbon, Chory has pioneered the development of plants that not only help mitigate CO2 levels in the atmosphere but also improve soil quality and increase crop yields. Her passion for combating climate change through plant science underscores her belief in a collective global effort to address this worldwide challenge. Read more about her .
“Dr. Chory is one of the leading figures in modern plant biology research. Her lab has laid the foundation of multiple important research fields.”
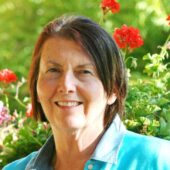
Virginia Walbot Development: Reproduction
Dr. Virginia Walbot and her team have been researching maize anther development since 2005. Notably, they demonstrated that the initial step in cell fate determination involves hypoxia triggering the specification of archesporial cells, which subsequently determine neighboring cells as somatic in anther primordia. They elucidated the roles of small secreted proteins and discovered the involvement of reproductive phasiRNAs at specific stages and in particular cell types. By investigating a wide range of cell fate mutants, they have systematically organized the timeline of steps in anther ontogeny. Recently, utilizing single-cell RNA-seq, they have meticulously documented the stages from archesporial cell development through to pollen maturation and the differentiation of each somatic cell type.
“Excellent scientist and teacher.”
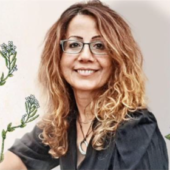
Alessandra Devoto Genes & Genomes: Gene regulatory networks & Signal Transduction
Professor Alessandra Devoto, a distinguished scientist with a deep-seated passion for plant molecular biology, has developed a significant career since completing her education at the University of Rome Sapienza, in Italy. After earning her BSc and MSc, she pursued a PhD in Plant Molecular Biology, which fueled her lifelong commitment to research. Since 2006, Prof. Devoto has been an influential figure at Royal Holloway University of London, where she leads groundbreaking research on plant responses to environmental stressors with an aim to enhance plant yield and adaptation. Her work, particularly in the area of jasmonates and their role in plant stress and defense mechanisms, has led to vital insights into plant growth regulation under stress conditions. Prof. Devoto has contributed extensively to the understanding of molecular pathways that can improve stress responses and has developed novel methods for analyzing phytopharmaceuticals and enhancing microbial processing of biomass. In addition to her research excellence, she is an acknowledged mentor of the next generation of scientists.
“Prof. Devoto’s tireless dedication to advancing plant stress biology and her unwavering commitment to nurturing the growth of young scientists have left an indelible mark.” “Like a sturdy oak, Prof. Devoto stands tall, providing shade and shelter to those under her mentorship. Her legacy is not merely in research papers or accolades but in the flourishing minds she has cultivated.”
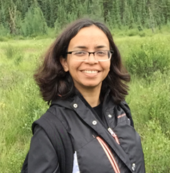
Sona Pandey Signal Transduction & Hormone Biology
Dr. Sona Pandey’s group focuses on how plants impact and respond to their surroundings using examples from species ranging from mosses and model plants such as Arabidopsis to crops such as soybean and sorghum. At the mechanistic level, the group studies a class of conserved signaling proteins, the heterotrimeric G-proteins (G-proteins) which control developmental and signaling pathways regulating stress responses and yield in plants. In addition to being an accomplished researcher, Sona has a reputation in and beyond her lab as an excellent mentor. She led the Danforth Center REU program for many years, mentoring undergraduates annually and increasing DEI in the field of plant sciences.
“Sona has been a tireless leader, establishing and overseeing an excellent NSF-REU program, mentoring students, advancing equity, inclusion, and women’s issues and pursuing impactful science.” “Sona is one of the most compassionate and knowledgeable mentors I have been fortunate to work with.” “I wish to become a scientist and a human like Dr. Pandey.” “Dr. Pandey’s courage in facing adversity has been an inspiration for me and others to pursue our goals.”
Mid-Career Researchers
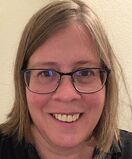
Carolyn Rasmussen Cell Biology: General
Research in the Rasmussen lab is focused on understanding how plant cell divisions are oriented and how division plane positioning influences plant growth and development. In addition, Dr. Rasmussen is committed to mentoring and providing research opportunities to students historically excluded from STEM. She directs the undergraduate REU Summer program at the University of California-Riverside. In addition, Dr. Rasmussen has contributed to the plant biology community through service on several committees and membership in external scientific societies.
“Dr. Rasmussen is an excellent researcher and highly deserves recognition for inspiring others.”
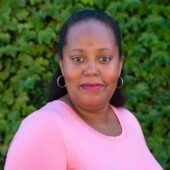
Tessa Burch-Smith Cell Biology
Dr. Tessa Burch-Smith has made significant strides in the field of plant biology, particularly in understanding intercellular communication through plasmodesmata in plant cells. Her research has expanded the knowledge base of how plasmodesmata function. Dr. Burch-Smith’s recent scholarly contributions include exploring the role of plasmodesmata in defense responses in Nicotiana benthamiana, as well as investigating the mechanisms of redox regulation in cell-to-cell communication, particularly in the context of viral spread within plant tissues. Each of these studies not only advances our understanding of plant biology but also underscores the complexity and importance of intercellular communication in plant resilience and adaptation. Beyond her research, Dr. Burch-Smith is lauded for her commitment to mentoring, particularly focused on supporting underrepresented minorities and individuals with disabilities in STEM fields. Her service to the plant biology community is extensive, including roles such as chair of the Science Policy Committee of ASPB, and membership on various editorial boards and conference organizing committees. This well-rounded professional portfolio showcases Dr. Burch-Smith as a dedicated scientist and mentor, making her a distinguished figure in plant biology and an exemplary leader.
“For her groundbreaking work in the field of plasmodesmata and her tireless efforts to make the pursuit of scientific careers accessible to all.”
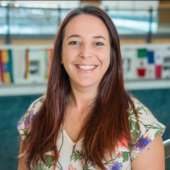
Patricia Baldrich Genes & Genomes: Gene Regulation & Transcriptional Networks
Dr. Patricia Baldrich’s work focuses on small RNAs in plants, ranging from creating atlases of small RNAs in potatoes to detecting them in rice samples and identifying their presence in Arabidopsis apoplastic fluid. She investigates their role in gene expression regulation and plant development, with implications for biotechnology in hybrid plant improvement. At the core of Patricia’s research is the quest to unravel the mechanisms governing gene expression regulation and plant development through the lens of small RNAs.
“Dr. Baldrich opened my mind to the career possibilities in science that I had never considered before. She is incredibly dedicated to making sure those around her are promoted with her, and is an amazing advocate for her mentees and employees.”
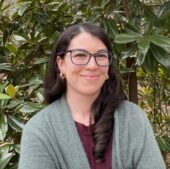
Rachel Egger Development: Reproduction
Rachel Egger is an incredible researcher and mentor, her publication and patent record is stellar, and she leads the Reproductive Biology team at Syngenta seeds research. Recent studies by Egger and colleagues have explored innovative techniques such as cyto-swapping post haploid induction, which is critical for developing elite, transformable haploid inducers in maize. Other research delves into the application of a pathogen Trojan horse approach to manipulate maize anther cell behavior, offering insights into the cellular mechanisms underlying pre-meiotic anther development and male fertility, and establishing a framework for evaluating developmental defects at the cellular level, using both morphological and molecular data across various maize anther mutants. She has mentored students at Maize Meetings for several years and continues to connect Academia with Industry. She is a constant advocate for others.
“Rachel is a stellar researcher and mentor, connecting industry with academia and advancing women in the workplace.”
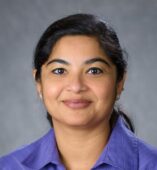
Aardra Kachroo Biotic Interactions: Plant-Microbe Immunity
Dr. Aardra Kachroo’s research aims to understand the interconnections between primary metabolic and defense signaling pathways while addressing immediate problems in agriculture. She utilizes a combination of host plants and pathogens to do both. The current focus in her lab is to understand the molecular underpinnings of a specific systemic signaling mechanism called systemic acquired resistance (SAR). When activated, SAR provides broad spectrum immunity at the whole plant level and can exhibit limited transgenerational transmission as well as to neighboring individuals. Her group is studying the overlap between SAR signaling with another recently identified root-shoot-root signaling mechanism that regulates the associations between legumes and nitrogen-fixing rhizobacteria. In addition to her research, Aardra Kachroo is both an advocate for and a mentor to others. She serves on the board of the Kentucky Association of Professional African American Women, and she served as President of the Bluegrass Indo-American Society, and as a Program Director at the National Science Foundation. She also leads a biotech company that develops sustainable and environmentally friendly pest management products for plants.
“Aardra Kachroo’s research at the University of Kentucky focuses on unraveling the molecular mechanisms of plant immunity. Using molecular biology and genomics, she explores how plants recognize and defend against pathogens, offering insights crucial for enhancing crop protection and global food security.” “I am privileged to receive guidance from a remarkable woman who has truly been a beacon of inspiration on my journey.” “Aardra serves selflessly to empower Women in Science and Plant Biology”
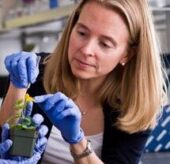
Carrie Wu Whole-Plant: Environmental & Eco physiological
Dr. Carrie Wu is a plant evolutionary ecologist who studies how the environment shapes the genetic structure and evolutionary trajectory of natural plant populations. Her research interests broadly encompass plant evolutionary ecology, local adaptation in natural plant populations, the invasion trajectory of introduced species, hybridization, and plant responses to a rapidly changing climate.
“Dr. Carrie Wu is a phenomenal role model and mentor. She was instrumental in shaping my perspective of science and nurturing my curiosity.” “Dr. Wu remains a large reason I had the courage to pursue a career in plant biology as a female scientist.”
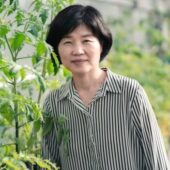
Jeongim Kim Biochemistry: Specialized Metabolites
Dr. Jeongim Kim is an assistant professor in the Horticultural Sciences Department and a member of the Plant Molecular and Cellular Biology (PMCB) graduate program. She earned her PhD at Purdue University, focusing on auxin biosynthesis and plant abiotic stress physiology. During her post-doctoral tenure at Purdue, she delved into phenylpropanoid biosynthesis, contributing significantly to several projects aimed at enhancing biofuel production. Dr. Kim’s research encompasses a broad spectrum, from the nutritional value of muscadine genotypes to the modulation of phenolic compounds in various plant varieties. Her recent investigations shed light on topics such as aldoxime metabolism, antioxidant capacity in lettuce, and the spatio-temporal control of phenylpropanoid biosynthesis. With a wealth of knowledge and a dedication to advancing plant science, Dr. Kim continues to make impactful contributions to her chosen field, plant natural products, and their abilities to enhance nutritional value or plant resilience.
“Dr. Jeongim Kim has established herself as an outstanding scientist while remaining unflinchingly kind and compassionate with her mentees. She mentored brilliantly these students throughout their PhD and led them toward successful careers in academia.”
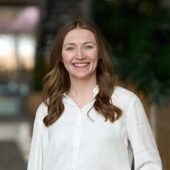
Katie Murphy Phenomics
Dr. Katherine Murphy, a Principal Investigator and Director of Phenotyping at the Donald Danforth Plant Science Center, uses high-throughput, image-based phenotyping to study how plants respond to environmental stressors, with a focus on maize and heat stress.
“What I admire most about Katie is her remarkable ability to communicate science exceptionally well. She skillfully conveys scientific concepts on multiple platforms that reach beyond the plant science community.”
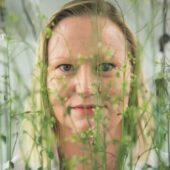
Lucia Strader Development: Vegetative
Dr. Lucia Strader’s research delves into the intricacies of normal plant morphogenesis and environmental responses, which necessitate the integration of multiple signals to modulate auxin responsiveness, biosynthesis, transport, and storage forms. As a critical plant hormone, auxin governs both cell division and expansion, thereby orchestrating numerous developmental events and environmental responses. Dr. Strader’s investigations are centered around gaining a molecular understanding of auxin signaling, auxin homeostasis, and the crosstalk between auxin and other hormones. By unraveling these molecular intricacies, her research aims to provide insight into the strategies employed by plants to adapt their growth in response to various environmental factors.
“Lucia is an incredible mentor and researcher who has made exemplary contributions to the auxin biology field.”

Ru Zhang Abiotic: Light & Temperature
Dr. Ru Zhang’s research is dedicated to understanding how plants respond to high temperatures by studying green algae, with a particular focus on photosynthesis. Dr. Zhang’s lab investigates how high temperatures impact photosynthesis, aiming to identify the most heat-sensitive components of this process. Currently, her research employs Chlamydomonas, an excellent model organism for understanding how photosynthetic cells react to elevated temperatures. With the growing threat of climate change exacerbating heat stress on crops, Dr. Zhang’s work holds significant importance in enhancing crop heat tolerance, ultimately contributing to global food security. Additionally, Dr. Zhang is recognized for her mentorship and leadership qualities, actively fostering the growth and development of individuals in her lab. Beyond her lab, Dr. Zhang actively contributes to various organizations at the Danforth Center, including the International Women in Science Hub.
“Ru Zhang is an excellent mentor that truly goes above and beyond to build up the people in her lab.” “Dr. Zhang has great leadership qualities in addition to being a passionate plant science researcher. She is a role model.”
Early Career Researchers
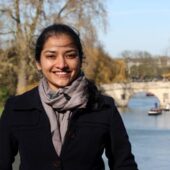
Pallavi Singh Biochemistry: Photosynthesis & BioEnergy
Since September 2022, Pallavi has been a Lecturer at the University of Essex (UK). Her research group focuses on studying various aspects of water use strategies in crop plants. The Singh lab aims to enhance photosynthetic productivity by concentrating on the complementary requirement to balance plant water supply with carbon gain, maximizing water use efficiency. The overarching goal of their research is to develop more water-efficient crops and promote climate-smart agriculture in the face of changing climate. Pallavi Singh has revolutionized the understanding of plant grafting by discovering a pluripotent tissue that enables grafting in monocotyledons like bananas and oil palms, which can help combat diseases and reduce deforestation. Her research on C4 photosynthesis has identified how C4 genes incorporate light-responsive elements, potentially enabling the enhancement of crop yields through genetic engineering. Singh has also found significant intraspecific variation in C4 traits within Gynandropsis gynandra, paving the way for the introduction of these traits into C3 crops. Additionally, her work has elucidated the MPK3-SUB1A1 module’s role in rice submergence tolerance, offering critical insights for developing flood-resistant rice varieties.
“Originating from modest beginnings, she began her research journey in India, and now stands as a paragon of research excellence and a source of inspiration for women of color in the UK academic landscape.”

Seema Sahay Biochemistry: Photosynthesis & BioEnergy
Dr. Seema Sahay is an exceptional researcher whose career trajectory showcases resilience and dedication. She earned her degree at Aligarh Muslim University and subsequently served as an assistant professor in Mexico before transitioning to the United States, where she continued her research endeavors amidst the challenges posed by the pandemic. Dr. Sahay’s notable contributions include mapping genes that govern variation in photosynthetic performance within plant populations, shedding light on crucial mechanisms underlying plant adaptation to environmental stressors. Her research encompasses diverse areas, including genetic control of photoprotection, modulation of gene expression under stress conditions, and the effects of biostimulants on plant resilience. Dr. Sahay’s work significantly advances our understanding of plant biology and holds promise for enhancing crop performance and resilience in the face of changing environmental conditions.
“Dr. Sahay has overcome language, cultural, economic, and religious barriers to move forward our understanding of how natural genetic diversity governs photosynthesis in plants.”
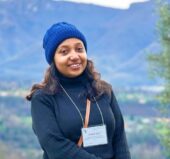
Sreya Das Abiotic: Light & Temperature
Sreya Das is deeply engaged in investigating the intricate crosstalk between temperature and light signals and its impact on the regulation of plant growth and development, with a primary focus on utilizing Arabidopsis as a model organism. Her research interests span a wide range of areas, including light and temperature signaling in plants, stress biology, plant developmental biology, and epigenetics. Through her studies, Dr. Das explores how plants modulate gene expression to influence essential processes such as cell elongation, flowering time, hormone metabolism, photoreceptor sensing, and overall yield under diverse light and temperature conditions, offering valuable insights for enhancing crop performance. Furthermore, Dr. Das’s contributions extend beyond her scientific endeavors. As a senior PhD student, she has demonstrated exemplary dedication, passion, and innovation, earning recognition as the ‘Best Collaborator’ within her lab. Additionally, Sreya actively engages in volunteer work and holds leadership positions in student-led organizations focused on community outreach and empowerment, exemplifying her commitment to making a positive impact beyond academia.
“Nurturing not only seeds, but women in plant biologists also develop roots of scientific discoveries and their contributions that blossom into scientific understanding and enriching a brighter future for all.” “I am fortunate to have a colleague whose dedication, passion, and innovation embody the spirit of inspiring women.”
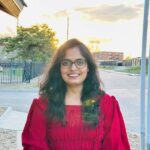
Surbhi Kumawat Genes & Genomes: Genome Editing
Surbhi Kumawat is a plant functional geneticist whose career focuses on the molecular basis of plant traits through the integration of quantitative genetics and genome editing. Her early work during graduate studies explored the molecular mechanisms of silicon uptake in plants. Currently, she is delving into the evolutionary dynamics of plant telomeres, using Mimulus as a model to understand why plant telomeric sequences diverge significantly from the conserved sequences found in vertebrates and Arabidopsis. Her research has also contributed significantly to genomic studies, as evidenced by her publications on topics ranging from the role of aquaporins in nutrient transport and stress responses to the implications of telomerase RNA transposition in telomere evolution. Through her work, Surbhi aims to innovate agricultural practices by developing resilient and high-yielding crop varieties, contributing to sustainable food systems and global food security.
“Surbhi has done very impressive research so far at such a young age.” “In addition to her research, which is impressive, Surbhi is also a contributor to her community.”
____________________________
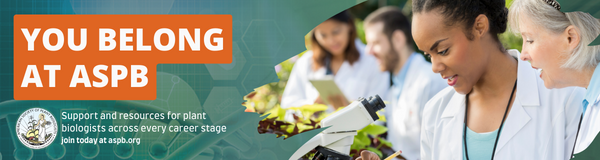
3 thoughts on “25 Inspiring Women in Plant Biology”
Thanks for sharing this list of inspiring colleagues. Very refreshing. One can’t, however, fail to notice the total lack of anyone in plant systematics, a symptom of the progressive abandonment of organismal biology in our academic instructions, which we will one day regret.
Agreed; I had the same thoughts…, slightly broader framing, to include evolutionary biology in general
Regarding the comments of Pete Lowry and Jonathan Wendel: we agree! As the committee selected from nominees only, we could not broaden beyond the areas recommended. The Women in Plant Biology Committee encourages all disciplines to nominate their inspiring women colleagues when we re-host this event. In the meantime, please feel free to add kudos to your female plant biology colleagues in the comments!
Leave a Comment Cancel reply
Information
- Author Services
Initiatives
You are accessing a machine-readable page. In order to be human-readable, please install an RSS reader.
All articles published by MDPI are made immediately available worldwide under an open access license. No special permission is required to reuse all or part of the article published by MDPI, including figures and tables. For articles published under an open access Creative Common CC BY license, any part of the article may be reused without permission provided that the original article is clearly cited. For more information, please refer to https://www.mdpi.com/openaccess .
Feature papers represent the most advanced research with significant potential for high impact in the field. A Feature Paper should be a substantial original Article that involves several techniques or approaches, provides an outlook for future research directions and describes possible research applications.
Feature papers are submitted upon individual invitation or recommendation by the scientific editors and must receive positive feedback from the reviewers.
Editor’s Choice articles are based on recommendations by the scientific editors of MDPI journals from around the world. Editors select a small number of articles recently published in the journal that they believe will be particularly interesting to readers, or important in the respective research area. The aim is to provide a snapshot of some of the most exciting work published in the various research areas of the journal.
Original Submission Date Received: .
- Active Journals
- Find a Journal
- Proceedings Series
- For Authors
- For Reviewers
- For Editors
- For Librarians
- For Publishers
- For Societies
- For Conference Organizers
- Open Access Policy
- Institutional Open Access Program
- Special Issues Guidelines
- Editorial Process
- Research and Publication Ethics
- Article Processing Charges
- Testimonials
- Preprints.org
- SciProfiles
- Encyclopedia
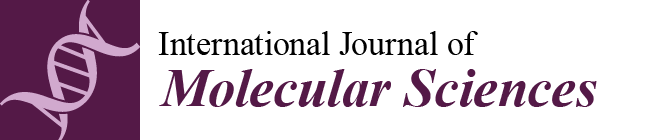
Article Menu
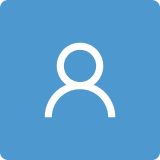
- Subscribe SciFeed
- Recommended Articles
- Google Scholar
- on Google Scholar
- Table of Contents
Find support for a specific problem in the support section of our website.
Please let us know what you think of our products and services.
Visit our dedicated information section to learn more about MDPI.
JSmol Viewer
Unveiling the secrets of oil palm genetics: a look into omics research, 1. introduction, 2. oil palm genomics, 2.1. oil palm genome sequencing, 2.2. research on molecular markers for important traits in oil palm.
Molecular Markers | Important Traits | References |
---|---|---|
SNP, SSR | oil content | [ ] |
SSR | hull thickness | [ ] |
SNP | stem height | [ ] |
SSR | drought-tolerant | [ ] |
SSR; | drought-tolerant | [ ] |
SNP | drought-tolerant | [ ] |
SSR | illegitimate progenies | [ ] |
SSR | identification of QTLs in oil palm | [ ] |
SSR | cold tolerance | [ ] |
SSR | identification of QTLs associated with callogenesis and embryogenesis in oil palm | [ ] |
EST-SSR | fruit exocarp colour | [ ] |
RFLP | genotypic discrimination | [ ] |
SNP | fatty acid content | [ ] |
SNP | height of oil palm | [ ] |
Intron polymorphisms (IP) | oil palm fatty acid composition | [ ] |
SSR | genetic diversity | [ , , ] |
SSR | sex ratio | [ ] |
SNP | vitamin E biosynthesis | [ ] |
SNP-CAPS | fruit traits | [ ] |
2.3. Gene Resource Mining and Function Analysis of Important Traits in Oil Palm
Gene Family | Function | Reference |
---|---|---|
Abiotic stress | ||
CBF | Increased tolerance to low temperatures | [ ] |
ABA | Enhance resistance to drought | [ ] |
bZIP | Highly expressed under low temperature, salinity, and drought stress conditions | [ ] |
AP2/ERF | Increased tolerance to salt, low temperatures, and drought | [ ] |
HRE2(ERF-VII) | HRE2 responds to flood-induced hypoxia | [ ] |
MYB | Increased tolerance to stresses such as salinity, cold, and drought | [ ] |
WRKY | Involved in abiotic stress responses such as drought, salinity, and heat | [ ] |
EgSPCH(bHLH) | EgSPCH produces more pores in response to salt stress | [ ] |
ARF | EgARF responds to abiotic stresses (cold, drought, and salt stress) | [ ] |
CAT | EgCAT enhances tolerance to low temperature, drought, and salt stress | [ ] |
Biotic stress | ||
CNL R gene | Expression in the early stages of oil palm defence mechanisms | [ ] |
AGLU1 RCH10 | Increasing oil palm resistance towards G. boninense | [ ] |
LCC | EgLCC24 gene detects oil palm seedlings resistant to G. boninense | [ ] |
Fatty acids | ||
MADS-box | Fruit development and oil accumulation | [ ] |
MAD | Mediating medium-chain fatty acid (MCFA) anabolism in oil palm mesocarp | [ ] |
GDSL | Enhanced lipid content | [ ] |
ACBP | Lipid accumulation | [ ] |
Others | ||
CAD | Lignin biosynthesis | [ ] |
3. Oil Palm Transcriptomics
Trait for Transcriptome Analysis | Transcription Factor | Summary | Reference |
---|---|---|---|
Somatic embryogenesis | FTIP, FRIGIDA-LIKE, and NF-YA, | The reproductive time of plants may be related to somatic embryogenesis potential | [ ] |
Drought | MYBs, HOXs, NF-Ys | A potential miRNA target gene for oil palm under water deficit conditions | [ ] |
Pi-deficiency | PHL7, NIP6–1 and 14–3-3 | Involved in the lack of regulation and domestication of oil palm Pi | [ ] |
Waterlogging | LBD41, HRA1, HRE2 | Respond to waterlogging | [ ] |
High somatic embryogenesis rate | LE, DDX28, HD-ZIP, NPF | The physiological state related to somatic embryogenesis potential was revealed | [ ] |
Bud Rot Disease | Clon34 and Clon57 | Control pathogen | [ ] |
Defoliation stress | / | Elucidating the mechanism of oil palm sex determination. | [ ] |
Bagworm stress | P450, GST and CCE AChE, nAChR, ACCase, GABA, RyR | Insecticide targets for detoxification and modification potential | [ ] |
Aluminium stress | DREB1F, NAC | Induces the expression of internal detoxification enzymes | [ ] |
Water deprivation | NF-YA3, HOX32, GRF1 | It plays a certain role in the response of oil palm seedlings to drought and salt stress. | [ ] |
Salt stress | MYC, G-Box, ABRE, TATA-box | Using identified genes to try to mitigate salt stress | [ ] |
G. boninense infection | target gene #5, #13, #16, #6 and #15, #1, #9, #12, and #14 | It may be related to early infection reaction to G. boninense | [ ] |
4. Oil Palm Proteomics
5. oil palm metabolomics, 6. conclusions and outlook, author contributions, conflicts of interest.
- Damayant, S.; Andry, S.; Khairurrijal; Kartasasmita, R.E. Isolation of β-carotene from Palm ( Elaeis guineensis Jacq.) Oil Using Transesterification-adsorption-desorption Method and its Characterization. J. Appl. Sci. 2014 , 14 , 2615–2621. [ Google Scholar ] [ CrossRef ]
- Babu, B.K.; Mathur, R.K.; Anitha, P.; Ravichandran, G.; Bhagya, H.P. Phenomics, genomics of oil palm ( Elaeis guineensis Jacq.): Way forward for making sustainable and high yielding quality oil palm. Physiol. Mol. Biol. Plants 2021 , 27 , 587–604. [ Google Scholar ] [ CrossRef ] [ PubMed ]
- Singh, R.; Ong-Abdullah, M.; Low, E.-T.L.; Manaf, M.A.A.; Rosli, R.; Nookiah, R.; Ooi, L.C.-L.; Ooi, S.E.; Chan, K.-L.; Halim, M.A.; et al. Oil palm genome sequence reveals divergence of interfertile species in Old and New worlds. Nature 2013 , 500 , 335–339. [ Google Scholar ] [ CrossRef ] [ PubMed ]
- Sundram, K.; Sambanthamurthi, R.; Tan, Y.A. Palm fruit chemistry and nutrition. Asia Pac. J. Clin. Nutr. 2003 , 12 , 355–362. [ Google Scholar ]
- Mba, O.I.; Dumont, M.-J.; Ngadi, M. Palm Oil: Processing, Characterization and Utilization in the Food Industry—A Review. Food Biosci. 2015 , 10 , 26–41. [ Google Scholar ] [ CrossRef ]
- Nainggolan, M.; Sinaga, A.G. Characteristics of fatty acid composition and minor constituents of red palm olein and palm kernel oil combination. J. Adv. Pharm. Technol. Res. 2021 , 12 , 22–26. [ Google Scholar ] [ CrossRef ]
- Barcelos, E.; Rios Sde, A.; Cunha, R.N.; Lopes, R.; Motoike, S.Y.; Babiychuk, E.; Skirycz, A.; Kushnir, S. Oil palm natural diversity and the potential for yield improvement. Front. Plant Sci. 2015 , 6 , 190. [ Google Scholar ] [ CrossRef ]
- Rival, A.; Beule, T.; Barre, P.; Hamon, S.; Duval, Y.; Noirot, M. Comparative flow cytometric estimation of nuclear DNA content in oil palm ( Elaeis guineensis Jacq) tissue cultures and seed-derived plants. Plant Cell Rep. 1997 , 16 , 884–887. [ Google Scholar ] [ CrossRef ] [ PubMed ]
- Pellicer, J.; Leitch, I.J. The Plant DNA C-values database (release 7.1): An updated online repository of plant genome size data for comparative studies. New Phytol. 2020 , 226 , 301–305. [ Google Scholar ] [ CrossRef ]
- Jin, J.; Lee, M.; Bai, B.; Sun, Y.; Qu, J.; Rahmadsyah; Alfiko, Y.; Lim, C.H.; Suwanto, A.; Sugiharti, M.; et al. Draft genome sequence of an elite Dura palm and whole-genome patterns of DNA variation in oil palm. DNA Res. 2016 , 23 , 527–533. [ Google Scholar ] [ CrossRef ]
- Utomo, C.; Tanjung, Z.A.; Aditama, R.; Buana, R.F.N.; Pratomo, A.D.M.; Tryono, R.; Liwang, T. Draft Genome Sequence of the Phytopathogenic Fungus Ganoderma boninense , the Causal Agent of Basal Stem Rot Disease on Oil Palm. Am. Soc. Microbiol. 2018 , 6 . [ Google Scholar ] [ CrossRef ] [ PubMed ]
- Ong, A.-L.; Teh, C.-K.; Mayes, S.; Massawe, F.; Appleton, D.R.; Kulaveerasingam, H. An Improved Oil Palm Genome Assembly as a Valuable Resource for Crop Improvement and Comparative Genomics in the Arecoideae Subfamily. Plants 2020 , 9 , 1476. [ Google Scholar ] [ CrossRef ] [ PubMed ]
- Mohd Sanusi, N.S.N.; Rosli, R.; Chan, K.-L.; Halim, M.A.A.; Ting, N.-C.; Singh, R.; Low, E.-T.L. Integrated consensus genetic map and genomic scaffold re-ordering of oil palm ( Elaeis guineensis ) genome. Comput. Biol. Chem. 2023 , 102 , 107801. [ Google Scholar ] [ CrossRef ] [ PubMed ]
- Sanusi, N.S.N.M.; Rosli, R.; Halim, M.A.A.; Chan, K.-L.; Nagappan, J.; Azizi, N.; Amiruddin, N.; Tatarinova, T.V.; Low, E.-T.L. PalmXplore: Oil palm gene database. Database 2018 , 2018 , bay095. [ Google Scholar ] [ CrossRef ] [ PubMed ]
- Uthaipaisanwong, P.; Chanprasert, J.; Shearman, J.R.; Sangsrakru, D.; Yoocha, T.; Jomchai, N.; Jantasuriyarat, C.; Tragoonrung, S.; Tangphatsornruang, S. Characterization of the chloroplast genome sequence of oil palm ( Elaeis guineensis Jacq.). Gene 2012 , 500 , 172–180. [ Google Scholar ] [ CrossRef ] [ PubMed ]
- Jaligot, E.; Rival, A.; Beulé, T.; Dussert, S.; Verdeil, J.L. Somaclonal variation in oil palm ( Elaeis guineensis Jacq.): The DNA methylation hypothesis. Plant Cell Rep. 2000 , 19 , 684–690. [ Google Scholar ] [ CrossRef ]
- Low, E.T.; Rosli, R.; Jayanthi, N.; Mohd-Amin, A.H.; Azizi, N.; Chan, K.L.; Maqbool, N.J.; Maclean, P.; Brauning, R.; McCulloch, A.; et al. Analyses of hypomethylated oil palm gene space. PLoS ONE 2014 , 9 , e86728. [ Google Scholar ] [ CrossRef ] [ PubMed ]
- Ong-Abdullah, M.; Ordway, J.M.; Jiang, N.; Ooi, S.-E.; Kok, S.-Y.; Sarpan, N.; Azimi, N.; Hashim, A.T.; Ishak, Z.; Rosli, S.K.; et al. Loss of Karma transposon methylation underlies the mantled somaclonal variant of oil palm. Nature 2015 , 525 , 533–537. [ Google Scholar ] [ CrossRef ] [ PubMed ]
- Sarpan, N.; Taranenko, E.; Ooi, S.-E.; Low, E.-T.L.; Espinoza, A.; Tatarinova, T.V.; Ong-Abdullah, M. DNA methylation changes in clonally propagated oil palm. Plant Cell Rep. 2020 , 39 , 1219–1233. [ Google Scholar ] [ CrossRef ]
- Ooi, S.-E.; Choo, C.-N.; Sarpan, N.; Wong, C.-K.; Wong, W.-C. Karma - EgDEF1 methylation in Elaeis guineensis clonal mother palms that produced high mantling rates in the second clonal generation. Vitr. Cell. Dev. Biol. Plant 2024 , 60 , 176–182. [ Google Scholar ] [ CrossRef ]
- Billotte, N.; Marseillac, N.; Risterucci, A.M.; Adon, B.; Brottier, P.; Baurens, F.C.; Singh, R.; Herrán, A.; Asmady, H.; Billot, C.; et al. Microsatellite-based high density linkage map in oil palm ( Elaeis guineensis Jacq.). Theor. Appl. Genet. 2005 , 110 , 754–765. [ Google Scholar ] [ CrossRef ] [ PubMed ]
- Jaligot, E.; Beulé, T.; Rival, A. Methylation-sensitive RFLPs: Characterisation of two oil palm markers showing somaclonal variation-associated polymorphism. Theor. Appl. Genet. 2002 , 104 , 1263–1269. [ Google Scholar ] [ CrossRef ] [ PubMed ]
- Singh, R.; Cheah, S.C. Analysis of the inheritance of AFLP markers in an interspecific cross of oil palm using the pseudo-testcross strategy. J. Oil Palm Res. 1999 , 11 , 64–73. [ Google Scholar ]
- Mayes, S.; Jack, P.L.; Corley, R.H.; Marshall, D.F. Construction of a RFLP genetic linkage map for oil palm ( Elaeis guineensis Jacq.). Genome 1997 , 40 , 116–122. [ Google Scholar ] [ CrossRef ] [ PubMed ]
- Mohan, M.; Nair, S.; Bhagwat, A.; Krishna, T.G.; Yano, M.; Bhatia, C.R.; Sasaki, T. Genome mapping, molecular markers and marker-assisted selection in crop plants. Mol. Breed. 1997 , 3 , 87–103. [ Google Scholar ] [ CrossRef ]
- Ho, C.-L.; Kwan, Y.-Y.; Choi, M.-C.; Tee, S.-S.; Ng, W.-H.; Lim, K.-A.; Lee, Y.-P.; Ooi, S.-E.; Lee, W.-W.; Tee, J.-M.; et al. Analysis and functional annotation of expressed sequence tags (ESTs) from multiple tissues of oil palm ( Elaeis guineensis Jacq.). BMC Genom. 2007 , 8 , 381. [ Google Scholar ] [ CrossRef ]
- Zhao, H.; Zhang, T.; Meng, X.; Song, J.; Zhang, C.; Gao, P. Genetic Mapping and QTL Analysis of Fruit Traits in Melon ( Cucumis melo L.). Curr. Issues Mol. Biol. 2023 , 45 , 3419–3433. [ Google Scholar ] [ CrossRef ]
- Cardona, C.C.C.; Coronado, Y.M.; Conronado, A.C.M.; Ochoa, I.J.B. Genetic diversity in oil palm ( Elaeis guineensis Jacq) using RAM (Random Amplified Microsatellites). Bragantia 2018 , 77 , 546–556. [ Google Scholar ] [ CrossRef ]
- Sunilkumar, K.; Murugesan, P.; Mathur, R.K.; Rajesh, M.K. Genetic diversity in oil palm ( Elaeis guineensis and Elaeis oleifera ) germplasm as revealed by microsatellite (SSR) markers. Indian J. Agric. Sci. 2020 , 90 , 741–745. [ Google Scholar ] [ CrossRef ]
- Suraninpong, P.; Nuanlaong, S. Comparative transcriptome profiling and molecular marker development for oil palm fruit color. Sci. Rep. 2022 , 12 , 15507. [ Google Scholar ] [ CrossRef ]
- Mohd Shaha, F.R.; Liew, P.L.; Qamaruz Zaman, F.; Nulit, R.; Barin, J.; Rolland, J.; Yong, H.Y.; Boon, S.H. Genotyping by sequencing for the construction of oil palm ( Elaeis guineensis Jacq.) genetic linkage map and mapping of yield related quantitative trait loci. PeerJ 2024 , 12 , e16570. [ Google Scholar ] [ CrossRef ] [ PubMed ]
- Kwong, Q.B.; Teh, C.K.; Ong, A.L.; Heng, H.Y.; Lee, H.L.; Mohamed, M.; Low, J.Z.-B.; Apparow, S.; Chew, F.T.; Mayes, S.; et al. Development and Validation of a High-Density SNP Genotyping Array for African Oil Palm. Mol. Plant 2016 , 9 , 1132–1141. [ Google Scholar ] [ CrossRef ] [ PubMed ]
- Babu, B.K.; Mathur, R.K.; Ravichandran, G.; Anita, P.; Venu, M.V.B. Genome wide association study (GWAS) and identification of candidate genes for yield and oil yield related traits in oil palm ( Eleaeis guineensis ) using SNPs by genotyping-based sequencing. Genomics 2020 , 112 , 1011–1020. [ Google Scholar ] [ CrossRef ] [ PubMed ]
- Wibowo, C.S.; Apriyanto, A.; Ernawan, R.; Neing, D.; Susilo, R.; Cordell, H.J.; Gatehouse, A.M.R.; Edwards, M.G. Genetic variants associated with leaf spot disease resistance in oil palm ( Elaeis guineensis ): A genome-wide association study. Plant Pathol. 2023 , 72 , 1626–1636. [ Google Scholar ] [ CrossRef ]
- Yaakub, Z.; Kamaruddin, K.; Singh, R.; Mustafa, S.; Marjuni, M.; Ting, N.C.; Amiruddin, M.D.; Leslie, L.E.; Cheng-Li, O.L.; Sritharan, K.; et al. An integrated linkage map of interspecific backcross 2 (BC(2)) populations reveals QTLs associated with fatty acid composition and vegetative parameters influencing compactness in oil palm. BMC Plant Biol. 2020 , 20 , 356. [ Google Scholar ] [ CrossRef ] [ PubMed ]
- Zhou, L.; Yarra, R.; Zhao, Z.; Jin, L.; Cao, H. Development of SSR markers based on transcriptome data and association mapping analysis for fruit shell thickness associated traits in oil palm ( Elaeis guineensis Jacq.). 3 Biotech 2020 , 10 , 280. [ Google Scholar ] [ CrossRef ] [ PubMed ]
- Lee, M.; Xia, J.H.; Zou, Z.; Ye, J.; Rahmadsyah; Alfiko, Y.; Jin, J.; Lieando, J.V.; Purnamasari, M.I.; Lim, C.H.; et al. A consensus linkage map of oil palm and a major QTL for stem height. Sci. Rep. 2015 , 5 , 8232. [ Google Scholar ] [ CrossRef ] [ PubMed ]
- Nuanlaong, S.; Wuthisuthimethavee, S.; Azzeme, A.M.; Suraninpong, P. Optimized Method for the Identification of Candidate Genes and Molecular Maker Development Related to Drought Tolerance in Oil Palm ( Elaeis guineensis Jacq.). Plants 2022 , 11 , 2317. [ Google Scholar ] [ CrossRef ]
- Suraninpong, P.; Thongkhao, K.; Azzeme, A.M.; Suksa-Ard, P. Monitoring Drought Tolerance in Oil Palm: Choline Monooxygenase as a Novel Molecular Marker. Plants 2023 , 12 , 3089. [ Google Scholar ] [ CrossRef ]
- Zolkafli, S.H.; Ithnin, M.; Chan, K.-L.; Zainol Abidin, M.I.; Ismail, I.; Ting, N.C.; Ooi, L.C.-L.; Singh, R. Optimal set of microsatellite markers required to detect illegitimate progenies in selected oil palm ( Elaeis guineensis Jacq.) breeding crosses. Breed. Sci. 2021 , 71 , 253–260. [ Google Scholar ] [ CrossRef ]
- Bhagya, H.P.; Kalyana Babu, B.; Gangadharappa, P.M.; Naika, M.B.N.; Satish, D.; Mathur, R.K. Identification of QTLs in oil palm ( Elaeis guineensis Jacq.) using SSR markers through association mapping. J. Genet. 2020 , 99 , 19. [ Google Scholar ] [ CrossRef ] [ PubMed ]
- Zulfugarov, I.S.; Tovuu, A.; Eu, Y.J.; Dogsom, B.; Poudyal, R.S.; Nath, K.; Hall, M.; Banerjee, M.; Yoon, U.C.; Moon, Y.H.; et al. Production of superoxide from Photosystem II in a rice ( Oryza sativa L.) mutant lacking PsbS. BMC Plant Biol. 2014 , 14 , 242. [ Google Scholar ] [ CrossRef ] [ PubMed ]
- Ting, N.C.; Jansen, J.; Nagappan, J.; Ishak, Z.; Chin, C.W.; Tan, S.G.; Cheah, S.C.; Singh, R. Identification of QTLs associated with callogenesis and embryogenesis in oil palm using genetic linkage maps improved with SSR markers. PLoS ONE 2013 , 8 , e53076. [ Google Scholar ] [ CrossRef ] [ PubMed ]
- Jack, P.L.; Dimitrijevic, T.A.F.; Mayes, S. Assessment of nuclear, mitochondrial and chloroplast RFLP markers in oil palm ( Elaeis guineensis Jacq.). Theor. Appl. Genet. 1995 , 90 , 643–649. [ Google Scholar ] [ CrossRef ]
- Xia, W.; Luo, T.; Dou, Y.; Zhang, W.; Mason, A.S.; Huang, D.; Huang, X.; Tang, W.; Wang, J.; Zhang, C.; et al. Identification and Validation of Candidate Genes Involved in Fatty Acid Content in Oil Palm by Genome-Wide Association Analysis. Front. Plant Sci. 2019 , 10 , 1263. [ Google Scholar ] [ CrossRef ]
- Somyong, S.; Phetchawang, P.; Bihi, A.K.; Sonthirod, C.; Kongkachana, W.; Sangsrakru, D.; Jomchai, N.; Pootakham, W.; Tangphatsornruang, S. A SNP variation in an expansin ( EgExp4 ) gene affects height in oil palm. PeerJ 2022 , 10 , e13046. [ Google Scholar ] [ CrossRef ]
- Li, J.; Yang, Y.; Sun, X.; Liu, R.; Xia, W.; Shi, P.; Zhou, L.; Wang, Y.; Wu, Y.; Lei, X.; et al. Development of Intron Polymorphism Markers and Their Association with Fatty Acid Component Variation in Oil Palm. Front. Plant Sci. 2022 , 13 , 885418. [ Google Scholar ] [ CrossRef ]
- Myint, K.A.; Yaakub, Z.; Rafii, M.Y.; Oladosu, Y.; Samad, M.Y.A.; Ramlee, S.I.; Mustaffa, S.; Arolu, F.; Abdullah, N.; Marjuni, M.; et al. Genetic Diversity Assessment of MPOB-Senegal Oil Palm Germplasm Using Microsatellite Markers. BioMed Res. Int. 2021 , 2021 , 6620645. [ Google Scholar ] [ CrossRef ] [ PubMed ]
- Zaki, N.M.; Singh, R.; Rosli, R.; Ismail, I. Elaeis oleifera Genomic-SSR Markers: Exploitation in Oil Palm Germplasm Diversity and Cross-Amplification in Arecaceae. Int. J. Mol. Sci. 2012 , 13 , 4069–4088. [ Google Scholar ] [ CrossRef ]
- Ting, N.C.; Zaki, N.M.; Rosli, R.; Low, E.T.; Ithnin, M.; Cheah, S.C.; Tan, S.G.; Singh, R. SSR mining in oil palm EST database: Application in oil palm germplasm diversity studies. J. Genet. 2010 , 89 , 135–145. [ Google Scholar ] [ CrossRef ]
- Somyong, S.; Poopear, S.; Sunner, S.K.; Wanlayaporn, K.; Jomchai, N.; Yoocha, T.; Ukoskit, K.; Tangphatsornruang, S.; Tragoonrung, S. ACC oxidase and miRNA 159a, and their involvement in fresh fruit bunch yield (FFB) via sex ratio determination in oil palm. Mol. Genet. Genom. 2016 , 291 , 1243–1257. [ Google Scholar ] [ CrossRef ] [ PubMed ]
- Dou, Y.; Xia, W.; Mason, A.S.; Huang, D.; Sun, X.; Fan, H.; Xiao, Y. Developing functional markers for vitamin E biosynthesis in oil palm. PLoS ONE 2021 , 16 , e0259684. [ Google Scholar ] [ CrossRef ]
- Venu, M.V.B.; Babu, B.K. Identification of fruit forms using CAPS marker among the oil palm indigenous germplasm. J. Oilseeds Res. 2023 , 37 . [ Google Scholar ] [ CrossRef ]
- Ithnin, M.; Xu, Y.; Marjuni, M.; Serdari, N.M.; Amiruddin, M.D.; Low, E.-T.L.; Tan, Y.-C.; Yap, S.-J.; Ooi, L.C.L.; Nookiah, R.; et al. Multiple locus genome-wide association studies for important economic traits of oil palm. Tree Genet. Genomes 2017 , 13 , 103. [ Google Scholar ] [ CrossRef ]
- Ithnin, M.; Vu, W.T.; Shin, M.-G.; Suryawanshi, V.; Sherbina, K.; Zolkafli, S.H.; Serdari, N.M.; Amiruddin, M.D.; Abdullah, N.; Mustaffa, S.; et al. Genomic diversity and genome-wide association analysis related to yield and fatty acid composition of wild American oil palm. Plant Sci. 2021 , 304 , 110731. [ Google Scholar ] [ CrossRef ]
- Osorio-Guarín, J.A.; Garzón-Martínez, G.A.; Delgadillo-Duran, P.; Bastidas, S.; Moreno, L.P.; Enciso-Rodríguez, F.E.; Cornejo, O.E.; Barrero, L.S. Genome-wide association study (GWAS) for morphological and yield-related traits in an oil palm hybrid ( Elaeis oleifera × Elaeis guineensis) population. BMC Plant Biol. 2019 , 19 , 533. [ Google Scholar ] [ CrossRef ] [ PubMed ]
- Babu, B.K.; Mathur, R.K.; Ravichandran, G.; Venu, M.V.B. Genome-wide association study (GWAS) for stem height increment in oil palm ( Elaeis guineensis ) germplasm using SNP markers. Tree Genet. Genomes 2019 , 15 , 40. [ Google Scholar ] [ CrossRef ]
- Bhagya, H.P.; Kalyana Babu, B.; Mathur, R.K.; Ramajayam, D.; Ravichandran, G.; Anitha, P. Oil palm ( Elaeis guineensis Jacq.) germplasm genome-wide association analysis for the oil yield traits utilizing microsatellite markers. Ind. Crops Prod. 2024 , 218 , 118934. [ Google Scholar ] [ CrossRef ]
- Teh, C.-K.; Ong, A.-L.; Kwong, Q.-B.; Apparow, S.; Chew, F.-T.; Mayes, S.; Mohamed, M.; Appleton, D.; Kulaveerasingam, H. Genome-wide association study identifies three key loci for high mesocarp oil content in perennial crop oil palm. Sci. Rep. 2016 , 6 , 19075. [ Google Scholar ] [ CrossRef ]
- Babu, B.K.; Mathur, R.K.; Ravichandran, G.; Anitha, P.; Venu, M.V.B. Genome-wide association study for leaf area, rachis length and total dry weight in oil palm ( Eleaeis guineensis ) using genotyping by sequencing. PLoS ONE 2019 , 14 , e0220626. [ Google Scholar ] [ CrossRef ]
- Babu, K.; Mathur, R.K.; Venu, M.V.; Shil, S.; Ravichandran, G.; Anita, P.; Bhagya, H.P. Genome-wide association study (GWAS) of major QTLs for bunch and oil yield related traits in Elaeis guineensis L. Plant Sci. 2021 , 305 , 110810. [ Google Scholar ] [ CrossRef ]
- Teh, C.-K.; Muaz, S.D.; Tangaya, P.; Fong, P.-Y.; Ong, A.-L.; Mayes, S.; Chew, F.-T.; Kulaveerasingam, H.; Appleton, D. Characterizing haploinsufficiency of SHELL gene to improve fruit form prediction in introgressive hybrids of oil palm. Sci. Rep. 2017 , 7 , 3118. [ Google Scholar ] [ CrossRef ] [ PubMed ]
- Singh, R.; Low, E.-T.L.; Ooi, L.C.-L.; Ong-Abdullah, M.; Nookiah, R.; Ting, N.-C.; Marjuni, M.; Chan, P.-L.; Ithnin, M.; Manaf, M.A.A.; et al. The oil palm VIRESCENS gene controls fruit colour and encodes a R2R3-MYB . Nat. Commun. 2014 , 5 , 4106. [ Google Scholar ] [ CrossRef ] [ PubMed ]
- Chan, K.-L.; Tatarinova, T.V.; Rosli, R.; Amiruddin, N.; Azizi, N.; Halim, M.A.A.; Sanusi, N.S.N.M.; Jayanthi, N.; Ponomarenko, P.; Triska, M.; et al. Evidence-based gene models for structural and functional annotations of the oil palm genome. Biol. Direct 2017 , 12 , 21. [ Google Scholar ] [ CrossRef ]
- Zhang, Y.; Bai, B.; Lee, M.; Alfiko, Y.; Suwanto, A.; Yue, G.H. Cloning and characterization of EgGDSL , a gene associated with oil content in oil palm. Sci. Rep. 2018 , 8 , 11406. [ Google Scholar ] [ CrossRef ]
- Jin, Y.; Yuan, Y.; Gao, L.; Sun, R.; Chen, L.; Li, D.; Zheng, Y. Characterization and Functional Analysis of a Type 2 Diacylglycerol Acyltransferase ( DGAT2 ) Gene from Oil Palm ( Elaeis guineensis Jacq.) Mesocarp in Saccharomyces cerevisiae and Transgenic Arabidopsis thaliana . Front. Plant Sci. 2017 , 8 , 1791. [ Google Scholar ] [ CrossRef ] [ PubMed ]
- Rosli, R.; Chan, P.-L.; Chan, K.-L.; Amiruddin, N.; Low, E.-T.L.; Singh, R.; Harwood, J.L.; Murphy, D.J. In silico characterization and expression profiling of the diacylglycerol acyltransferase gene family (DGAT1, DGAT2, DGAT3 and WS/DGAT) from oil palm, Elaeis guineensis . Plant Sci. 2018 , 275 , 84–96. [ Google Scholar ] [ CrossRef ] [ PubMed ]
- Li, S.Y.; Zhang, Q.; Jin, Y.H.; Zou, J.X.; Zheng, Y.S.; Li, D.D. A MADS-box gene, EgMADS21 , negatively regulates EgDGAT2 expression and decreases polyunsaturated fatty acid accumulation in oil palm ( Elaeis guineensis Jacq.). Plant Cell Rep. 2020 , 39 , 1505–1516. [ Google Scholar ] [ CrossRef ]
- Chan, P.L.; Rose, R.J.; Abdul Murad, A.M.; Zainal, Z.; Ong, P.W.; Ooi, L.C.; Low, E.L.; Ishak, Z.; Yahya, S.; Song, Y.; et al. Early nodulin 93 protein gene: Essential for induction of somatic embryogenesis in oil palm. Plant Cell Rep. 2020 , 39 , 1395–1413. [ Google Scholar ] [ CrossRef ]
- Nizan, I.E.F.; Kamaruddin, K.; Ong, P.W.; Ramli, Z.; Singh, R.; Rose, R.J.; Chan, P.L. Overexpression of Oil Palm Early Nodulin 93 Protein Gene ( EgENOD93 ) Enhances In Vitro Shoot Regeneration in Arabidopsis thaliana. Mol. Biotechnol. 2022 , 64 , 743–757. [ Google Scholar ] [ CrossRef ]
- Tregear, J.W.; Richaud, F.; Collin, M.; Esbelin, J.; Parrinello, H.; Cochard, B.; Nodichao, L.; Morcillo, F.; Adam, H.; Jouannic, S. Micro-RNA-Regulated SQUAMOSA-PROMOTER BINDING PROTEIN-LIKE ( SPL ) Gene Expression and Cytokinin Accumulation Distinguish Early-Developing Male and Female Inflorescences in Oil Palm ( Elaeis guineensis ). Plants 2022 , 11 , 685. [ Google Scholar ] [ CrossRef ] [ PubMed ]
- Zhou, L.; Yarra, R. Genome-Wide Analysis of SPL /miR156 Module and Its Expression Analysis in Vegetative and Reproductive Organs of Oil Palm ( Elaeis guineensis ). Int. J. Mol. Sci. 2023 , 24 , 13658. [ Google Scholar ] [ CrossRef ] [ PubMed ]
- Li, J.; Yang, Y.; Iqbal, A.; Qadri, R.; Shi, P.; Wang, Y.; Wu, Y.; Fan, H.; Wu, G. Correlation analysis of cold-related gene expression with physiological and biochemical indicators under cold stress in oil palm. PLoS ONE 2019 , 14 , e0225768. [ Google Scholar ] [ CrossRef ]
- Wang, L.; Lee, M.; Ye, B.; Yue, G.H. Genes, pathways and networks responding to drought stress in oil palm roots. Sci. Rep. 2020 , 10 , 21303. [ Google Scholar ] [ CrossRef ]
- Zhou, L.; Yarra, R. Genome-wide identification and expression analysis of bZIP transcription factors in oil palm ( Elaeis guineensis Jacq.) under abiotic stress. Protoplasma 2022 , 259 , 469–483. [ Google Scholar ] [ CrossRef ]
- Zhou, L.; Yarra, R. Genome-Wide Identification and Characterization of AP2/ERF Transcription Factor Family Genes in Oil Palm under Abiotic Stress Conditions. Int. J. Mol. Sci. 2021 , 22 , 2821. [ Google Scholar ] [ CrossRef ] [ PubMed ]
- Lim, H.; Kobayashi, M.J.; Marsoem, S.N.; Irawati, D.; Kosugi, A.; Kondo, T.; Tani, N. Transcriptomic responses of oil palm ( Elaeis guineensis ) stem to waterlogging at plantation in relation to precipitation seasonality. Front. Plant Sci. 2023 , 14 , 1213496. [ Google Scholar ] [ CrossRef ]
- Zhou, L.; Yarra, R.; Yang, Y.; Liu, Y.; Yang, M.; Cao, H. The oil palm R2R3-MYB subfamily genes EgMYB111 and EgMYB157 improve multiple abiotic stress tolerance in transgenic Arabidopsis plants. Plant Cell Rep. 2022 , 41 , 377–393. [ Google Scholar ] [ CrossRef ]
- Lee, F.C.; Yeap, W.C.; Appleton, D.R.; Ho, C.-L.; Kulaveerasingam, H. Identification of drought responsive Elaeis guineensis WRKY transcription factors with sensitivity to other abiotic stresses and hormone treatments. BMC Genom. 2022 , 23 , 164. [ Google Scholar ] [ CrossRef ]
- Song, Z.; Wang, L.; Lai, C.; Lee, M.; Yang, Z.; Yue, G. EgSPEECHLESS Responses to Salt Stress by Regulating Stomatal Development in Oil Palm. Int. J. Mol. Sci. 2022 , 23 , 4659. [ Google Scholar ] [ CrossRef ]
- Jin, L.; Yarra, R.; Zhou, L.; Cao, H. The auxin response factor (ARF) gene family in Oil palm ( Elaeis guineensis Jacq.): Genome-wide identification and their expression profiling under abiotic stresses. Protoplasma 2022 , 259 , 47–60. [ Google Scholar ] [ CrossRef ] [ PubMed ]
- Zhou, L.; John Martin, J.J.; Li, R.; Zeng, X.; Wu, Q.; Li, Q.; Fu, D.; Li, X.; Liu, X.; Ye, J.; et al. Catalase (CAT) Gene Family in Oil Palm ( Elaeis guineensis Jacq.): Genome-Wide Identification, Analysis, and Expression Profile in Response to Abiotic Stress. Int. J. Mol. Sci. 2024 , 25 , 1480. [ Google Scholar ] [ CrossRef ] [ PubMed ]
- Rosli, R.; Amiruddin, N.; Ab Halim, M.A.; Chan, P.-L.; Chan, K.-L.; Azizi, N.; Morris, P.E.; Leslie Low, E.-T.; Ong-Abdullah, M.; Sambanthamurthi, R.; et al. Comparative genomic and transcriptomic analysis of selected fatty acid biosynthesis genes and CNL disease resistance genes in oil palm. PLoS ONE 2018 , 13 , e0194792. [ Google Scholar ] [ CrossRef ] [ PubMed ]
- Hanin, A.N.; Parveez, G.K.A.; Rasid, O.A.; Masani, M.Y.A. Biolistic-mediated oil palm transformation with alfalfa glucanase ( AGLU1 ) and rice chitinase ( RCH10 ) genes for increasing oil palm resistance towards Ganoderma boninense . Ind. Crops Prod. 2020 , 144 , 112008. [ Google Scholar ] [ CrossRef ]
- Faizah, R.; Putranto, R.A.; Raharti, V.R.; Supena, N.; Sukma, D.; Budiani, A.; Wening, S.; Sudarsono, S. Defense response changes in roots of oil palm ( Elaeis guineensis Jacq.) seedlings after internal symptoms of Ganoderma boninense Pat. infection. BMC Plant Biol. 2022 , 22 , 139. [ Google Scholar ] [ CrossRef ] [ PubMed ]
- Cao, J.; Wang, W.; Xu, X.; Li, S.-Y.; Zheng, Y.; Li, D.-D. Identification and Analysis of MADS-Box Genes Expressed in the Mesocarp of Oil Palm Fruit ( Elaeis guineensis Jacq.). Biochem. Genet. 2023 , 61 , 2382–2400. [ Google Scholar ] [ CrossRef ] [ PubMed ]
- Wang, Y.; Yan, J.; Yang, M.; Zou, J.; Zheng, Y.; Li, D. EgMADS3 directly regulates EgLPAAT to mediate medium-chain fatty acids (MCFA) anabolism in the mesocarp of oil palm. Plant Cell Rep. 2024 , 43 , 107. [ Google Scholar ] [ CrossRef ]
- Amiruddin, N.; Chan, P.-L.; Azizi, N.; Morris, P.E.; Chan, K.-L.; Ong, P.W.; Rosli, R.; Masura, S.S.; Murphy, D.J.; Sambanthamurthi, R.; et al. Characterization of Oil Palm Acyl-CoA-Binding Proteins and Correlation of Their Gene Expression with Oil Synthesis. Plant Cell Physiol. 2019 , 61 , 735–747. [ Google Scholar ] [ CrossRef ]
- Yusuf, C.Y.L.; Nabilah, N.S.; Taufik, N.; Seman, I.A.; Abdullah, M.P. Genome-wide analysis of the CAD gene family reveals two bona fide CAD genes in oil palm. 3 Biotech 2022 , 12 , 149. [ Google Scholar ] [ CrossRef ]
- Manzoni, C.; Kia, D.A.; Vandrovcova, J.; Hardy, J.; Wood, N.W.; Lewis, P.A.; Ferrari, R. Genome, transcriptome and proteome: The rise of omics data and their integration in biomedical sciences. Brief. Bioinform. 2016 , 19 , 286–302. [ Google Scholar ] [ CrossRef ]
- Yang, Z.; Liu, Z.; Xu, H.; Li, Y.; Huang, S.; Cao, G.; Shi, M.; Zhu, J.; Zhou, J.; Li, R.; et al. ArecaceaeMDB: A comprehensive multi-omics database for Arecaceae breeding and functional genomics studies. Plant Biotechnol. J. 2023 , 21 , 11–13. [ Google Scholar ] [ CrossRef ] [ PubMed ]
- Xiao, Y.; Xia, W.; Mason, A.S.; Cao, Z.; Fan, H.; Zhang, B.; Zhang, J.; Ma, Z.; Peng, M.; Huang, D. Genetic control of fatty acid composition in coconut ( Cocos nucifera ), African oil palm ( Elaeis guineensis ), and date palm ( Phoenix dactylifera ). Planta 2019 , 249 , 333–350. [ Google Scholar ] [ CrossRef ] [ PubMed ]
- Zhang, A.; Jin, L.; Yarra, R.; Cao, H.; Chen, P.; John Martin, J.J. Transcriptome analysis reveals key developmental and metabolic regulatory aspects of oil palm ( Elaeis guineensis Jacq.) during zygotic embryo development. BMC Plant Biol. 2022 , 22 , 112. [ Google Scholar ] [ CrossRef ] [ PubMed ]
- Apriyanto, A.; Compart, J.; Fettke, J. Transcriptomic analysis of mesocarp tissue during fruit development of the oil palm revealed specific isozymes related to starch metabolism that control oil yield. Front. Plant Sci. 2023 , 14 , 1220237. [ Google Scholar ] [ CrossRef ] [ PubMed ]
- Chu, K.L.; Koley, S.; Jenkins, L.M.; Bailey, S.R.; Kambhampati, S.; Foley, K.; Arp, J.J.; Morley, S.A.; Czymmek, K.J.; Bates, P.D.; et al. Metabolic flux analysis of the non-transitory starch tradeoff for lipid production in mature tobacco leaves. Metab. Eng. 2022 , 69 , 231–248. [ Google Scholar ] [ CrossRef ]
- Apriyanto, A.; Ernawan, R.; Putra, M.K.A.; Susilo, R.; Ajambang, W.; Pancoro, A.; Wibowo, C.S. Gene expression profiles of oil palm leaves from different oil yields and genetic population: Transcriptomic dataset. Data Brief 2023 , 48 , 109043. [ Google Scholar ] [ CrossRef ] [ PubMed ]
- Yang, M.; Yarra, R.; Zhang, R.; Zhou, L.; Jin, L.; Martin, J.J.J.; Cao, H. Transcriptome analysis of oil palm pistil during pollination and fertilization to unravel the role of phytohormone biosynthesis and signaling genes. Funct. Integr. Genom. 2022 , 22 , 261–278. [ Google Scholar ] [ CrossRef ]
- Zheng, Y.; Chen, C.; Liang, Y.; Sun, R.; Gao, L.; Liu, T.; Li, D. Genome-wide association analysis of the lipid and fatty acid metabolism regulatory network in the mesocarp of oil palm ( Elaeis guineensis Jacq.) based on small noncoding RNA sequencing. Tree Physiol. 2018 , 39 , 356–371. [ Google Scholar ] [ CrossRef ]
- Xia, W.; Dou, Y.; Liu, R.; Gong, S.; Huang, D.; Fan, H.; Xiao, Y. Genome-wide discovery and characterization of long noncoding RNAs in African oil palm ( Elaeis guineensis Jacq.). PeerJ 2020 , 8 , e9585. [ Google Scholar ] [ CrossRef ]
- Ooi, S.-E.; Feshah, I.; Nuraziyan, A.; Sarpan, N.; Ata, N.; Lim, C.-C.; Choo, C.-N.; Wong, W.-C.; Wong, F.-H.; Wong, C.-K.; et al. Leaf transcriptomic signatures for somatic embryogenesis potential of Elaeis guineensis . Plant Cell Rep. 2021 , 40 , 1141–1154. [ Google Scholar ] [ CrossRef ]
- Salgado, F.F.; da Silva, T.L.C.; Vieira, L.R.; Silva, V.N.B.; Leão, A.P.; Costa, M.; Togawa, R.C.; de Sousa, C.A.F.; Grynberg, P.; Souza, M.T., Jr. The early response of oil palm ( Elaeis guineensis Jacq.) plants to water deprivation: Expression analysis of miRNAs and their putative target genes, and similarities with the response to salinity stress. Front. Plant Sci. 2022 , 13 , 970113. [ Google Scholar ] [ CrossRef ] [ PubMed ]
- Kong, S.-L.; Abdullah, S.N.A.; Ho, C.-L.; Musa, M.H.b.; Yeap, W.-C. Comparative transcriptome analysis reveals novel insights into transcriptional responses to phosphorus starvation in oil palm ( Elaeis guineensis ) root. BMC Genom. Data 2021 , 22 , 6. [ Google Scholar ] [ CrossRef ] [ PubMed ]
- Sahara, A.; Roberdi, R.; Wiendi, N.M.A.; Liwang, T. Transcriptome profiling of high and low somatic embryogenesis rate of oil palm ( Elaeis guineensis Jacq. var. Tenera). Front. Plant Sci. 2023 , 14 , 1142868. [ Google Scholar ] [ CrossRef ] [ PubMed ]
- García-Gaona, M.; Botero-Rozo, D.; Araque, L.; Romero, H.M. The Dynamic Interaction between Oil Palm and Phytophthora palmivora in Bud Rot Disease: Insights from Transcriptomic Analysis and Network Modelling. J. Fungi 2024 , 10 , 164. [ Google Scholar ] [ CrossRef ] [ PubMed ]
- Apriyanto, A.; Ajambang, W. Transcriptomic dataset for early inflorescence stages of oil palm in response to defoliation stress. Data Brief 2022 , 41 , 107914. [ Google Scholar ] [ CrossRef ] [ PubMed ]
- Zainuddin, N.; Maidin, M.S.T.; Kamarudin, N.; Napiah, N.R.A.M.A.; Keni, M.F.; Masri, M.M.M. De novo transcriptome analysis of bagworm Metisa plana from highly infested oil palm estate in Perak revealed detoxification genes and potential insecticide targets. J. Asia Pac. Entomol. 2023 , 26 , 102039. [ Google Scholar ] [ CrossRef ]
- Mejia-Alvarado, F.S.; Botero-Rozo, D.; Araque, L.; Bayona, C.; Herrera-Corzo, M.; Montoya, C.; Ayala-Díaz, I.; Romero, H.M. Molecular network of the oil palm root response to aluminum stress. BMC Plant Biol. 2023 , 23 , 346. [ Google Scholar ] [ CrossRef ] [ PubMed ]
- Salgado, F.F.; Vieira, L.R.; Silva, V.N.B.; Leão, A.P.; Grynberg, P.; do Carmo Costa, M.M.; Togawa, R.C.; de Sousa, C.A.F.; Júnior, M.T.S. Expression analysis of miRNAs and their putative target genes confirm a preponderant role of transcription factors in the early response of oil palm plants to salinity stress. BMC Plant Biol. 2021 , 21 , 518. [ Google Scholar ] [ CrossRef ]
- Ferreira, T.M.M.; Ferreira Filho, J.A.; Leão, A.P.; de Sousa, C.A.F.; Souza, M.T., Jr. Structural and functional analysis of stress-inducible genes and their promoters selected from young oil palm ( Elaeis guineensis ) under salt stress. BMC Genom. 2022 , 23 , 735. [ Google Scholar ] [ CrossRef ]
- Faizah, R.; Putranto, R.A.; Wening, S.; Sukma, D.; Raharti, V.R.; Budiani, A.; Sudarsono, S.J.I.C.S.E.; Science, E. Differential expression of root specific genes of oil palm seedlings at early stage of Ganoderma boninense infection. IOP Conf. Ser. Earth Environ. Sci. 2020 , 418 , 012044. [ Google Scholar ] [ CrossRef ]
- Saand, M.A.; Li, J.; Wu, Y.; Zhou, L.; Cao, H.; Yang, Y. Integrative Omics Analysis of Three Oil Palm Varieties Reveals (Tanzania × Ekona) TE as a Cold-Resistant Variety in Response to Low-Temperature Stress. Int. J. Mol. Sci 2022 , 23 , 14926. [ Google Scholar ] [ CrossRef ] [ PubMed ]
- Silva Rde, C.; Carmo, L.S.; Luis, Z.G.; Silva, L.P.; Scherwinski-Pereira, J.E.; Mehta, A. Proteomic identification of differentially expressed proteins during the acquisition of somatic embryogenesis in oil palm ( Elaeis guineensis Jacq.). J. Proteom. 2014 , 104 , 112–127. [ Google Scholar ] [ CrossRef ] [ PubMed ]
- Tan, H.S.; Liddell, S.; Ong Abdullah, M.; Wong, W.C.; Chin, C.F. Differential proteomic analysis of embryogenic lines in oil palm ( Elaeis guineensis Jacq). J. Proteom. 2016 , 143 , 334–345. [ Google Scholar ] [ CrossRef ]
- Ribeiro, D.G.; de Almeida, R.F.; Fontes, W.; de Souza Castro, M.; de Sousa, M.V.; Ricart, C.A.O.; da Cunha, R.N.V.; Lopes, R.; Scherwinski-Pereira, J.E.; Mehta, A. Stress and cell cycle regulation during somatic embryogenesis plays a key role in oil palm callus development. J. Proteom. 2019 , 192 , 137–146. [ Google Scholar ] [ CrossRef ] [ PubMed ]
- Aroonluk, S.; Roytrakul, S.; Jantasuriyarat, C. Identification and Characterization of Phosphoproteins in Somatic Embryogenesis Acquisition during Oil Palm Tissue Culture. Plants 2020 , 9 , 36. [ Google Scholar ] [ CrossRef ] [ PubMed ]
- Balotf, S.; Wilson, R.; Tegg, R.S.; Nichols, D.S.; Wilson, C.R. Shotgun Proteomics as a Powerful Tool for the Study of the Proteomes of Plants, Their Pathogens, and Plant-Pathogen Interactions. Proteomes 2022 , 10 , 5. [ Google Scholar ] [ CrossRef ]
- Loei, H.; Lim, J.; Tan, M.; Lim, T.K.; Lin, Q.S.; Chew, F.T.; Kulaveerasingam, H.; Chung, M.C. Proteomic analysis of the oil palm fruit mesocarp reveals elevated oxidative phosphorylation activity is critical for increased storage oil production. J. Proteome Res. 2013 , 12 , 5096–5109. [ Google Scholar ] [ CrossRef ]
- Kok, S.-Y.; Namasivayam, P.; Ee, G.C.-L.; Ong-Abdullah, M. Comparative proteomic analysis of oil palm ( Elaeis guineensis Jacq.) during early fruit development. J. Proteom. 2021 , 232 , 104052. [ Google Scholar ] [ CrossRef ]
- Hassan, H.; Amiruddin, M.D.; Weckwerth, W.; Ramli, U.S. Deciphering key proteins of oil palm ( Elaeis guineensis Jacq.) fruit mesocarp development by proteomics and chemometrics. Electrophoresis 2019 , 40 , 254–265. [ Google Scholar ] [ CrossRef ]
- Rodrigues-Neto, J.C.; Correia, M.V.; Souto, A.L.; Ribeiro, J.A.d.A.; Vieira, L.R.; Souza, M.T.; Rodrigues, C.M.; Abdelnur, P.V. Metabolic fingerprinting analysis of oil palm reveals a set of differentially expressed metabolites in fatal yellowing symptomatic and non-symptomatic plants. Metabolomics 2018 , 14 , 142. [ Google Scholar ] [ CrossRef ]
- Neto, J.C.R.; Vieira, L.R.; de Aquino Ribeiro, J.A.; de Sousa, C.A.F.; Júnior, M.T.S.; Abdelnur, P.V. Metabolic effect of drought stress on the leaves of young oil palm ( Elaeis guineensis) plants using UHPLC–MS and multivariate analysis. Sci. Rep. 2021 , 11 , 18271. [ Google Scholar ] [ CrossRef ] [ PubMed ]
- Muhammad, I.I.; Abdullah, S.N.A.; Saud, H.M.; Shaharuddin, N.A.; Isa, N.M. The Dynamic Responses of Oil Palm Leaf and Root Metabolome to Phosphorus Deficiency. Metabolites 2021 , 11 , 217. [ Google Scholar ] [ CrossRef ] [ PubMed ]
- Santiago, K.A.A.; Wong, W.C.; Goh, Y.K.; Tey, S.H.; Ting, A.S.Y. Pathogenicity of monokaryotic and dikaryotic mycelia of Ganoderma boninense revealed via LC–MS-based metabolomics. Sci. Rep. 2024 , 14 , 5330. [ Google Scholar ] [ CrossRef ] [ PubMed ]
- Zakaria, L. Basal Stem Rot of Oil Palm: The Pathogen, Disease Incidence, and Control Methods. Plant Dis. 2023 , 107 , 603–615. [ Google Scholar ] [ CrossRef ] [ PubMed ]
- Neoh, B.K.; Teh, H.F.; Ng, T.L.; Tiong, S.H.; Thang, Y.M.; Ersad, M.A.; Mohamed, M.; Chew, F.T.; Kulaveerasingam, H.; Appleton, D.R. Profiling of metabolites in oil palm mesocarp at different stages of oil biosynthesis. J. Agric. Food Chem. 2013 , 61 , 1920–1927. [ Google Scholar ] [ CrossRef ]
- Rozali, N.L.; Tahir, N.I.; Hassan, H.; Othman, A.; Ramli, U.S. Identification of amines, amino and organic acids in oil palm ( Elaeis guineensis Jacq.) spear leaf using GC- and LC/Q-TOF MS metabolomics platforms. Biocatal. Agric. Biotechnol. 2021 , 37 , 102165. [ Google Scholar ] [ CrossRef ]
Click here to enlarge figure
Traits | Number of Accessions | Chromosomes | Number of QTLs | Number of Candidate Genes | Reference |
---|---|---|---|---|---|
compact fruit bunch | 422 | 4, 8, 11 | 4 | / | [ ] |
oil content | 196 | / | 33 | 55 | [ ] |
fatty acid composition; nutrient content | 210 | 2, 13 | 6 | 3 | [ ] |
oil content | 471 | 3, 5, 10, 13, 15 | / | / | [ ] |
disease resistance | 96 | 6, 7, 9 | 5 | / | [ ] |
leaf area size; high yield; | 115 | / | / | 5 | [ ] |
trunk height | 132 | 5, 6 | / | / | [ ] |
stem height | 96 | 1, 2, 4, 14, 16 | 7 | / | [ ] |
high oil production | 310 | 1, 4, 7, 10, 11, 12, 15 | 43 | / | [ ] |
The statements, opinions and data contained in all publications are solely those of the individual author(s) and contributor(s) and not of MDPI and/or the editor(s). MDPI and/or the editor(s) disclaim responsibility for any injury to people or property resulting from any ideas, methods, instructions or products referred to in the content. |
Share and Cite
Xu, W.; John Martin, J.J.; Li, X.; Liu, X.; Zhang, R.; Hou, M.; Cao, H.; Cheng, S. Unveiling the Secrets of Oil Palm Genetics: A Look into Omics Research. Int. J. Mol. Sci. 2024 , 25 , 8625. https://doi.org/10.3390/ijms25168625
Xu W, John Martin JJ, Li X, Liu X, Zhang R, Hou M, Cao H, Cheng S. Unveiling the Secrets of Oil Palm Genetics: A Look into Omics Research. International Journal of Molecular Sciences . 2024; 25(16):8625. https://doi.org/10.3390/ijms25168625
Xu, Wen, Jerome Jeyakumar John Martin, Xinyu Li, Xiaoyu Liu, Ruimin Zhang, Mingming Hou, Hongxing Cao, and Shuanghong Cheng. 2024. "Unveiling the Secrets of Oil Palm Genetics: A Look into Omics Research" International Journal of Molecular Sciences 25, no. 16: 8625. https://doi.org/10.3390/ijms25168625
Article Metrics
Article access statistics, further information, mdpi initiatives, follow mdpi.
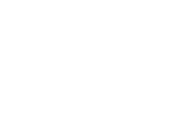
Subscribe to receive issue release notifications and newsletters from MDPI journals
- News by Country
- News by Industry
- Get News Alerts
- Press Releases
- About EIN Newsdesk
- How-to Videos
- EIN Newsdesk on Facebook
- EIN Newsdesk on Twitter
- News by U.S. State
- Agriculture
- Real Estate
- Create Your Own
- Submit Press Release
- Browse Featured Press Releases
- Browse All Press Releases
- Market Research Reports

Global Plant Genomics Market Report 2024: Trends, Strategies, And Opportunities

Plant Genomics Global Market Report 2024 – Market Size, Trends, And Global Forecast 2024-2033
The Business Research Company's Plant Genomics Global Market Report 2024 – Market Size, Trends, And Global Forecast 2024-2033
LONDON, GREATER LONDON, UK, August 8, 2024 / EINPresswire.com / -- The global plant genomics market is set to grow from $10.21 billion in 2023 to $11.12 billion in 2024, reflecting a compound annual growth rate (CAGR) of 8.9%. Despite challenges, the market is anticipated to reach $15.72 billion by 2028 at a CAGR of 9.0%, driven by advancements in genomic research, crop yield optimization, and increasing demand for sustainable agriculture.
Growing Demand for Food Productivity Drives Market Growth The demand for enhanced food productivity is a primary factor fueling the growth of the plant genomics market. By analyzing plant genetics, scientists can develop crops that yield more food per unit of land. Recent data from the Food and Agriculture Organization highlights significant increases in global cereal and wheat production, underscoring the role of plant genomics in addressing global food security and productivity challenges.
Explore comprehensive insights into the global plant genomics market with a detailed sample report: https://www.thebusinessresearchcompany.com/sample_request?id=10216&type=smp
Key Players and Technological Advancements Major players in the plant genomics market include Agilent Technologies Inc., Illumina Inc., Eurofins Scientific SE, and NRGene Ltd., among others. These companies are focused on technological advancements to enhance their market presence. For example, Nucleome Informatics Pvt. Ltd. recently launched the NKC Center for genomic research, advancing their capabilities in genome sequencing and research.
Trends Shaping the Future Key trends in the plant genomics market include the adoption of CRISPR and genome editing technologies, advancements in sequencing, and the integration of omics technologies. These innovations are transforming how genetic information is utilized to improve crop traits and overall agricultural efficiency.
Market Segments • Type: Molecular Engineering, Genetic Engineering, Genome Editing • Trait: Yield Improvement, Disease Resistance, Herbicide Tolerance • Technology: DNA And RNA Sequencing, Genotyping, Marker-Assisted Selection (MAS), Bioinformatics • Application: Cereals And Grains, Oilseeds And Pulses, Fruits And Vegetables
Geographical Insights: Asia-Pacific Leading the Market Asia-Pacific emerged as the largest region in the plant genomics market in 2023 and is expected to continue leading in the forecast period. The region’s strong research capabilities and investments in agricultural technologies contribute to its dominance in the market.
Explore the report store to make a direct purchase of the report https://www.thebusinessresearchcompany.com/report/plant-genomics-global-market-report
Plant Genomics Global Market Report 2024 from TBRC covers the following information: • Market size data for the forecast period: Historical and Future • Market analysis by region: Asia-Pacific, China, Western Europe, Eastern Europe, North America, USA, South America, Middle East and Africa. • Market analysis by countries: Australia, Brazil, China, France, Germany, India, Indonesia, Japan, Russia, South Korea, UK, USA.
Trends, opportunities, strategies and so much more. The Plant Genomics Global Market Report 2024 by The Business Research Company is the most comprehensive report that provides insights on plant genomics market size, plant genomics market drivers and trends, plant genomics market major players, competitors' revenues, market positioning, and market growth across geographies. The plant genomics market report helps you gain in-depth insights on opportunities and strategies. Companies can leverage the data in the report and tap into segments with the highest growth potential.
Browse Through More Similar Reports By The Business Research Company: Plant Growth Regulators Global Market Report 2024 https://www.thebusinessresearchcompany.com/report/plant-growth-regulators-global-market-report
Plant-Based Beverages Global Market Report 2024 https://www.thebusinessresearchcompany.com/report/plant-based-beverages-global-market-report
Agricultural Implement Global Market Report 2024 https://www.thebusinessresearchcompany.com/report/agricultural-implement-global-market-report
About The Business Research Company? The Business Research Company has published over 15000+ reports in 27 industries, spanning 60+ geographies. The reports draw on 1,500,000 datasets, extensive secondary research, and exclusive insights from interviews with industry leaders.
Global Market Model – Market Intelligence Database The Global Market Model, The Business Research Company’s flagship product, is a market intelligence platform covering various macroeconomic indicators and metrics across 60 geographies and 27 industries. The Global Market Model covers multi-layered datasets that help its users assess supply-demand gaps.
Contact Information The Business Research Company Europe: +44 207 1930 708 Asia: +91 8897263534 Americas: +1 315 623 0293
Oliver Guirdham The Business Research Company +44 20 7193 0708 email us here Visit us on social media: Facebook X LinkedIn YouTube
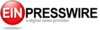
EIN Presswire does not exercise editorial control over third-party content provided, uploaded, published, or distributed by users of EIN Presswire. We are a distributor, not a publisher, of 3rd party content. Such content may contain the views, opinions, statements, offers, and other material of the respective users, suppliers, participants, or authors.
Biotechnology News Today by EIN Newsdesk & EIN Presswire (a press release distribution service)
Follow us on Facebook & Twitter and connect with us on LinkedIn
Newsmatics Inc. , 1025 Connecticut Avenue NW, Suite 1000, Washington, DC 20036 · Contact · About
© 1995-2024 Newsmatics Inc., a publisher of EIN News · All Rights Reserved · Privacy Policy · User Agreement
- Power Search
- All News Topics
- Technology News Topics
- Biotechnology News
- Biotechnology News Topics
- Biotechnology News by Country
- Biotechnology News by U.S. State
- World Media Directory
- Live Feed Wire
- 0 My Press Releases (0)
- Submit Release
- Browse Biotechnology Press Releases
- Browse Featured Releases
- Browse All Releases
- 0 My RSS Feeds (0)
- Create RSS Feed
- Search RSS Feeds
- Browse RSS Feeds
- Recently Visited News Feeds
- 0 My Newsletters (0)
- Create Newsletter
- Search Newsletters
- Browse Newsletters
- 0 My Events (0)
- Search Events
- Browse Events
- 0 My Topics (0)
- Add to My Topics
- Recently Visited
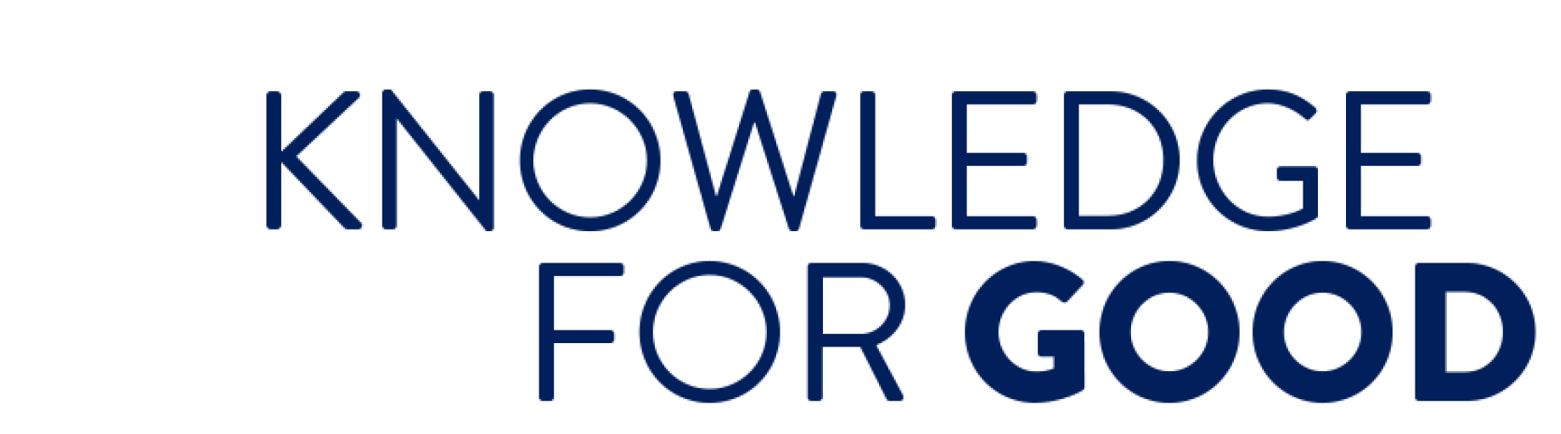
Digging into Native Plant Research
Support for research at the morris arboretum & gardens aims to advance our understanding of native plants.
In Pennsylvania, native plants, trees, and shrubs range from the Mountain Laurel—the state flower—to the American Chestnut, the Black-Eyed Susan, the Early Goldenrod, and many other species both common and rare.
These native plants are essential to the health and stability of our ecosystem, which in turn contributes to cleaner air and water, healthier soil, climate regulation, and a welcoming habitat for wildlife, including pollinators like bees and hummingbirds.
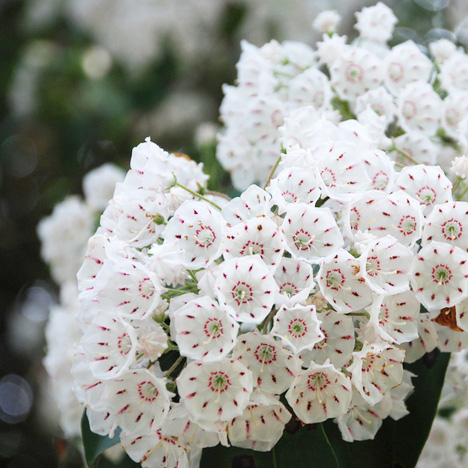
Mountain Laurel (pictured) is native to Pennsylvania. The Morris Arboretum & Gardens has been a hub for native plant research for nearly a century.
Timothy Block, PhD, the John J. Willaman Chair of Botany and Director of Plant Science at the Morris Arboretum & Gardens, has devoted his career as a botanist to studying native plants, overseeing a team that records any changes over time. “We don’t take a breath that isn’t supported by native plants,” says Block.
Despite their importance, many native species remain a mystery. “Pennsylvania is home to 55 species of native orchids,” says Block. “Unfortunately, many of them are critically imperiled and poorly understood biologically.” While people may rally to save endangered mammals like the once-scarce giant panda, Block says the same excitement doesn’t always translate to the plant world.
Jan Albaum, FA’84, GFA’87, understands the critical nature of native plants and shares Block’s enthusiasm for unlocking their mysteries. She and her husband, Harry Cerino, recently established the Jan Albaum and Harry Cerino Endowment for Native Plant Research in memory of Jan’s father, Martin Albaum.
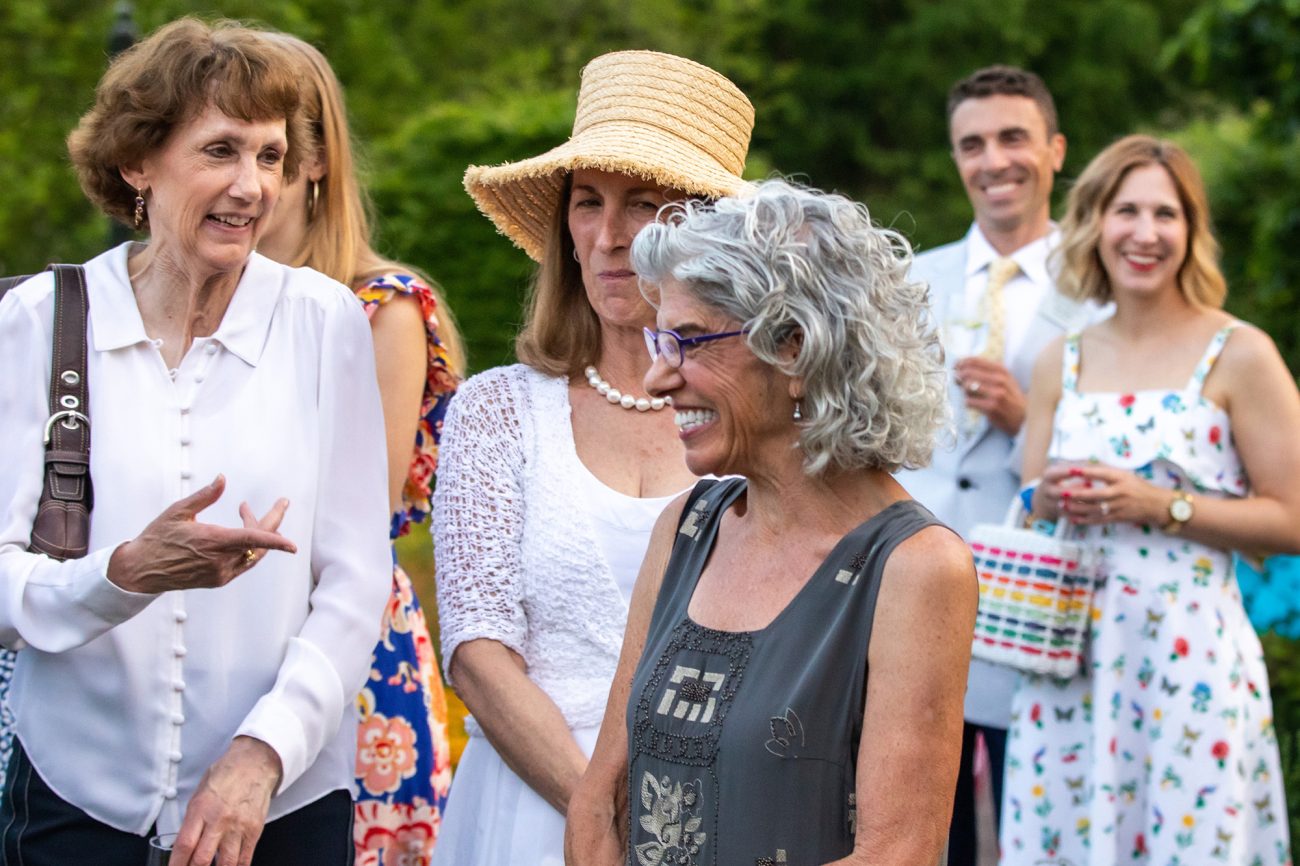
“The idea of investigating which plants do well in Pennsylvania as the climate changes really resonated with me,” says Albaum, who is a member of the Morris Arboretum & Garden’s Board of Advisors. In addition to the gift from Albaum and Cerino, the Morris is expanding its focus on native plants in other ways. They are set to break ground on a new plant science lab—a fundraising priority —in the fall of 2024, which will focus on regionally rare plant species and forest health. “The Morris has long been a leader in botanical science and this new molecular lab will take our scholarship to even greater heights,” says William Cullina, the F. Otto Haas Executive Director. “The lab will allow our researchers to conduct DNA and RNA extraction, going beneath the surface of what botanists see in the field to sequence their genetic code.”
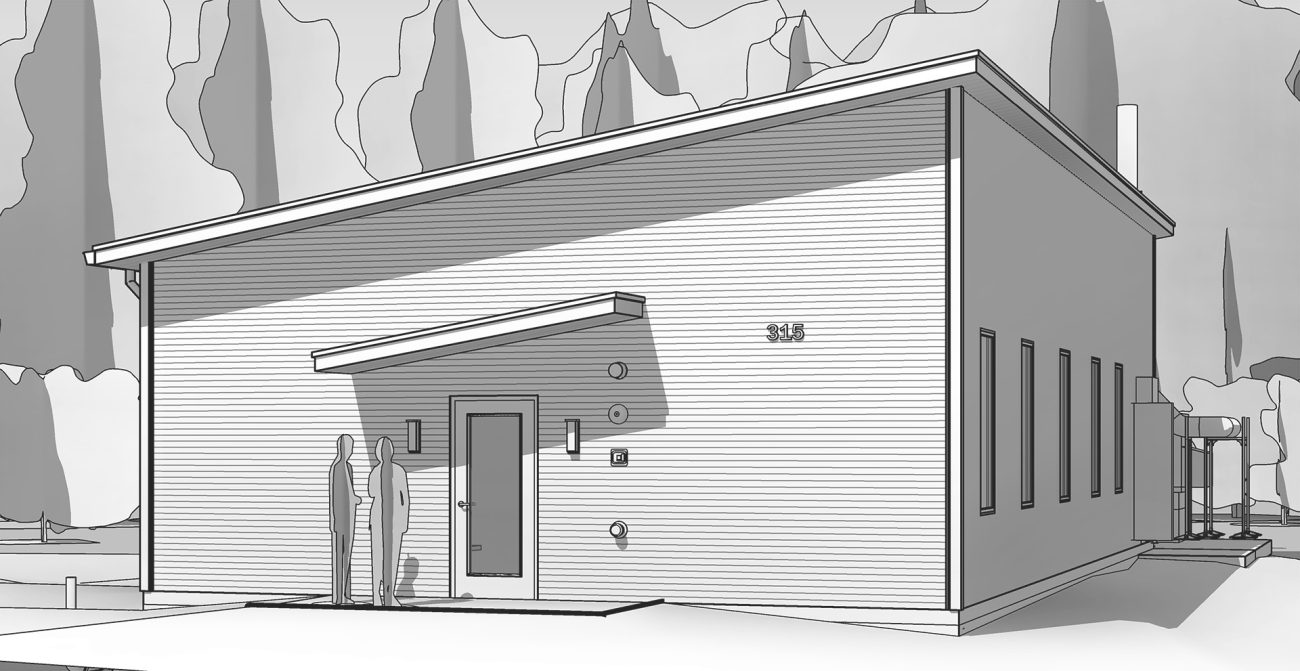
The new lab, combined with philanthropic support from Albaum and Cerino, will enable Block and his team to gain a deeper understanding of how native plants and climate change are intertwined. “Research doesn’t pay for itself, which is why people like Jan and Harry are so important to us,” says Block. “As botanists, we fight an uphill battle to find people who are concerned about what’s happening to plant communities over time. If plants disappear, we’re in big trouble.”
Support native plant research at the Morris
Related Stories & Media
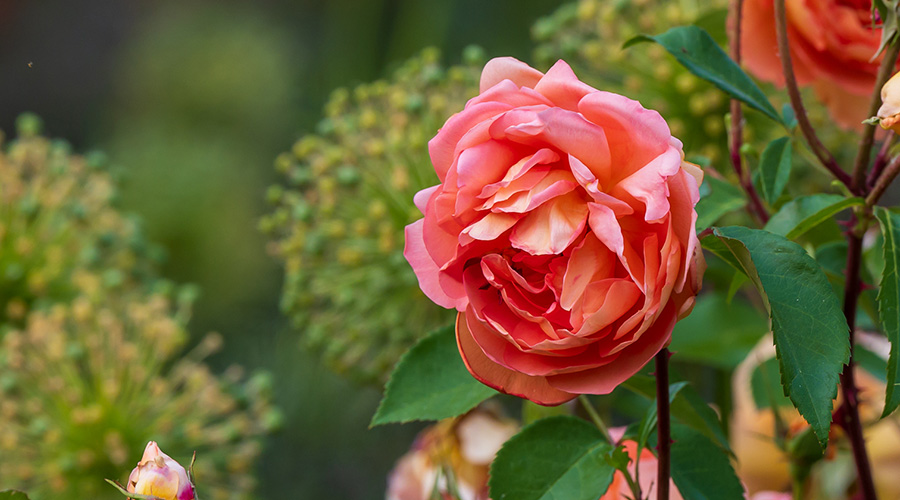
Stop and Smell the Roses
New renovations increase accessibility at the Morris Arboretum & Gardens' Rose Garden

On the Right Track
Garden Railway Gift to Establish a New “Chestnut Hill Station” at Morris Arboretum & Gardens
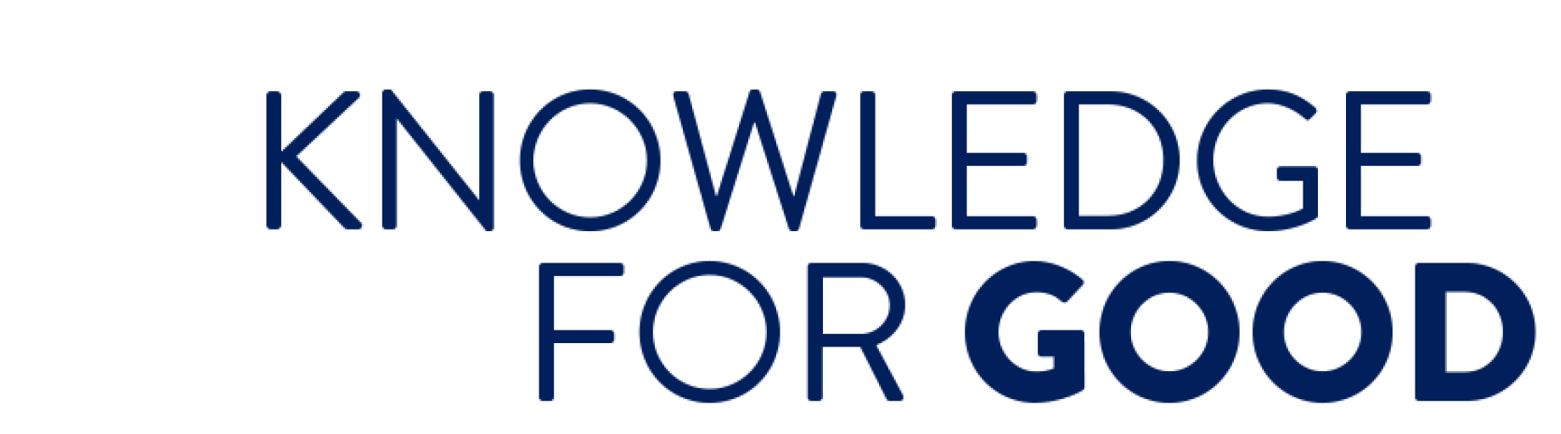
Pure Nature
The Wallace-Kane Wetlands at the Morris Arboretum & Gardens is a splendid sanctuary
EDITORIAL article
Editorial: plant chassis for synthetic biology and its application in biomanufacturing.
- 1 Key Laboratory of Engineering Biology for Low-carbon Manufacturing, Tianjin Institute of Industrial Biotechnology, Chinese Academy of Sciences, Tianjin, China
- 2 University of Chinese Academy of Sciences, Beijing, China
- 3 Tobacco Research Institute, Chinese Academy of Agricultural Sciences, Qingdao, China
- 4 Govind Ballabh Pant National Institute of Himalayan Environment, Almora, India
- 5 Department of Molecular Biology, Princeton University, Princeton, NJ, United States
- 6 Beijing Life Science Academy, Beijing, China
- 7 National Center of Technology Innovation for Synthetic Biology, Tianjin, China
Editorial on the Research Topic Plant chassis for synthetic biology and its application in biomanufacturing
In recent years, the global food and energy crises have attracted considerable attention. Plant synthetic biology is emerging as an attractive solution to these problems, by combining plant biology with engineering principles to design and produce new devices or products that are inexpensive and easy to scale up. Plant synthetic biology uses plants as a chassis to design and construct novel biological systems with specific functions or to produce valuable compounds through techniques such as gene editing and metabolic engineering. While a significant progress has been made in plant synthetic biology in the past few years, a comprehensive understanding of the underlying biosynthetic and regulatory mechanisms remains to be explored.
This research theme contains a collection of original research papers and reviews that collectively present the latest research trends and approaches on plant chassis and plant genes in green biomanufacturing, with the aim of facilitating the wider use of plant chassis materials in biomanufacturing and the development of plant synthetic biology. Here, we highlight several studies that aim to optimize the integration of metabolic pathways and plant chassis to produce valuable compound in a cost-effective manner. Various strategies are involved, including multi-omics analysis, chassis development and gene function studies.
Tobacco, a plant chassis, has been widely used as in vitro culture in plant synthetic biology. Thus, the study of metabolic network in its in vitro culture is of great significance. This can help to promote the application of in vitro technology in plant propagation. To gain a comprehensive understanding of the metabolic networks in the in vitro culture of tobacco, Yu et al. establish a genome-scale metabolic network (GSMN), a tool designed to facilitate the characterization of overall metabolic profiles. In comparison to soil-grown tobacco, in vitro tobacco exhibited slower growth, reduced biomass, inhibited photosynthesis, and altered metabolites and metabolic pathways.
Moringa oleifera and related species has potential applications in the health, food, cosmetic, and pharmaceutical industries. Klimek-Szczykutowicz et al. present a review, summarizing the application value of Moringa oleifera in terms of chemical composition, nutritional properties, pharmacological activity, cosmetic applications, and agronomic importance. This review also discusses feasibility of using Moringa oleifera as in vitro culture to develop plant micropropagation techniques and for agronomic application due to its inherent bactericidal and flocculating effects. These advances provide important insights for the progressive development of strategies for the application of moringa in various fields.
Buell et al. present a new plant chassis material, Populus . The authors summarized the strategy for creating new morphological populus chassis materials that alter the leaf-to-wood ratio of the tree by modifying trunk branching and tree height. These morphotypes can be modified into customized types that produce high-value biofuels, bioproducts, and biomaterials not only in specific organs but also in specific cell types. Taken together, these advances demonstrate that populus can be employed as a versatile feedstock and offer new opportunities for the application of genome engineering in crop species.
The plant cuticle consists of the polyester cutin, a common cuticular waxes, which are primarily a mixture of linear very long chain fatty acids (VLCFAs) and derivatives. Alexander et al. used the synthetic biology strategy to investigate whether the maize GL2 and GL2-LIKE proteins can influence the biosynthesis of VLCFA. They found that the maize glossy2 and glossy2-like genes encode BAHD acyltransferases and they may participate in the elongation of VLCFAs. Furthermore, Liza et al. investigate the VLCFA product profile in the strain with co-expression of GLOSSY2 or GLOSSY2-like and maize fatty acid elongase component enzymes on the VLCFA product profile. The results suggest that the apparent stimulatory role of GLOSSY2 or GLOSSY2-LIKE in enabling the synthesis of longer-chain VLCFAs in diploid yeast cells may be related to the mixing of plant enzyme components with the endogenous FAE complex.
In summary, the articles in this research theme address the impact of in vitro culture techniques on plant chassis metabolic networks, the development of novel plant chassis materials, and plant gene function. Plant chassis research will continue to address current challenges in synthetic biology and promote its application in biomanufacturing.
Author contributions
ZL: Conceptualization, Investigation, Writing – original draft, Writing – review & editing. ZZ: Writing – review & editing. SR: Writing – review & editing. LW: Writing – review & editing. PC: Writing – review & editing. LZ: Writing – original draft, Writing – review & editing.
The author(s) declare financial support was received for the research, authorship, and/or publication of this article. This work was supported by the National Key R&D Program of China (2022YFC3401800), the Hundred Talents Program of the Chinese Academy of Sciences (E3J56201), the Tianjin Synthetic Biotechnology Innovation Capacity Improvement Project (TSBICIP-CXRC-027; TSBICIP-IJCP-001; TSBICIP-IJCP-002) and Beijing Life Science Academy (2023000CB0040; 2023200CB0070).
Acknowledgments
We thank all the contributing authors and reviewers for their support in this Research Topic.
Conflict of interest
The authors declare that the research was conducted in the absence of any commercial or financial relationships that could be construed as a potential conflict of interest.
Publisher’s note
All claims expressed in this article are solely those of the authors and do not necessarily represent those of their affiliated organizations, or those of the publisher, the editors and the reviewers. Any product that may be evaluated in this article, or claim that may be made by its manufacturer, is not guaranteed or endorsed by the publisher.
Keywords: plant chassis, plant gene, synthetic biology, biomanufacturing, application
Citation: Liu Z, Zhang Z, Rawat S, Wang L, Cao P and Zhao L (2024) Editorial: Plant chassis for synthetic biology and its application in biomanufacturing. Front. Plant Sci. 15:1460378. doi: 10.3389/fpls.2024.1460378
Received: 06 July 2024; Accepted: 22 July 2024; Published: 01 August 2024.
Edited and Reviewed by:
Copyright © 2024 Liu, Zhang, Rawat, Wang, Cao and Zhao. This is an open-access article distributed under the terms of the Creative Commons Attribution License (CC BY) . The use, distribution or reproduction in other forums is permitted, provided the original author(s) and the copyright owner(s) are credited and that the original publication in this journal is cited, in accordance with accepted academic practice. No use, distribution or reproduction is permitted which does not comply with these terms.
*Correspondence: Peijian Cao, [email protected] ; Lei Zhao, [email protected]
Disclaimer: All claims expressed in this article are solely those of the authors and do not necessarily represent those of their affiliated organizations, or those of the publisher, the editors and the reviewers. Any product that may be evaluated in this article or claim that may be made by its manufacturer is not guaranteed or endorsed by the publisher.
Thank you for visiting nature.com. You are using a browser version with limited support for CSS. To obtain the best experience, we recommend you use a more up to date browser (or turn off compatibility mode in Internet Explorer). In the meantime, to ensure continued support, we are displaying the site without styles and JavaScript.
- View all journals
- Explore content
- About the journal
- Publish with us
- Sign up for alerts
- Review Article
- Published: 09 October 2013
Advanced genetic tools for plant biotechnology
- Wusheng Liu 1 ,
- Joshua S. Yuan 2 &
- C. Neal Stewart Jr 1 , 3
Nature Reviews Genetics volume 14 , pages 781–793 ( 2013 ) Cite this article
15k Accesses
155 Citations
12 Altmetric
Metrics details
- Plant biotechnology
- Plant genetics
A Corrigendum to this article was published on 26 November 2013
This article has been updated
There are several emerging genetic tools that will have increasingly important roles in the future of plant biotechnology and crop genetics.
Precise transgene or endogenous gene expression can be regulated at the transcriptional level by novel synthetic promoters, as well as synthetic transcriptional activators and repressors, for increased spatio-temporal control.
The recent development of several advanced DNA construction and assembly methods will allow the production of long DNA constructs and vectors that are needed for multigene transformation into plants.
Plant transformation with large constructs that are needed for metabolic pathway engineering is enabled by several techniques, including plant artificial chromosomes. There is no clear 'winner' among the several techniques presented.
Plant-genome editing using a host of new tools, including zinc-finger nucleases (ZFNs), transcription activator-like effector nucleases (TALENs) and clustered regularly interspaced short palindromic repeats (CRISPRs), is poised to have, perhaps, the greatest effect on precisely changing DNA sequences in crops in novel ways.
Tools for transgene removal and confinement are crucial for the commercialization of certain crops and crop–transgene combinations to ensure biosafety and government regulatory compliance.
Basic research has provided a much better understanding of the genetic networks and regulatory hierarchies in plants. To meet the challenges of agriculture, we must be able to rapidly translate this knowledge into generating improved plants. Therefore, in this Review, we discuss advanced tools that are currently available for use in plant biotechnology to produce new products in plants and to generate plants with new functions. These tools include synthetic promoters, 'tunable' transcription factors, genome-editing tools and site-specific recombinases. We also review some tools with the potential to enable crop improvement, such as methods for the assembly and synthesis of large DNA molecules, plant transformation with linked multigenes and plant artificial chromosomes. These genetic technologies should be integrated to realize their potential for applications to pressing agricultural and environmental problems.
This is a preview of subscription content, access via your institution
Access options
Subscribe to this journal
Receive 12 print issues and online access
176,64 € per year
only 14,72 € per issue
Buy this article
- Purchase on Springer Link
- Instant access to full article PDF
Prices may be subject to local taxes which are calculated during checkout
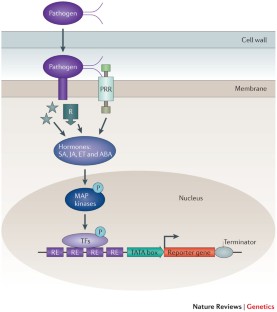
Similar content being viewed by others
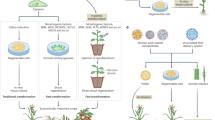
Recent advances in crop transformation technologies
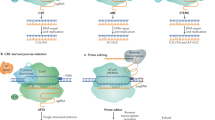
Applications of CRISPR–Cas in agriculture and plant biotechnology

Rewiring gene circuitry for plant improvement
Change history, 26 november 2013.
In Figure 4d, e of this article, the products of transgene excision and marker gene removal were shown incorrectly. The article has been corrected online. The authors apologize for the error.
Collins, N. C., Tardieu, F. & Tuberosa, R. Quantitative trait loci and crop performance under abiotic stress: where do we stand? Plant Physiol. 147 , 469–486 (2008).
CAS PubMed PubMed Central Google Scholar
Wollenweber, B., Porter, J. R. & Lubberstedt, T. Need for multidisciplinary research towards a second green revolution. Curr. Opin. Plant Biol. 8 , 337–341 (2005).
PubMed Google Scholar
Naqvi, S. et al. When more is better: multigene engineering in plants. Trends Plant Sci. 15 , 48–56 (2010).
CAS PubMed Google Scholar
Venter, M. Synthetic promoters: genetic control through cis engineering. Trends Plant Sci. 12 , 118–124 (2007).
De Wilde, C. et al. Plants as bioreactors for protein production: avoiding the problem of transgene silencing. Plant Mol. Biol. 43 , 347–359 (2000).
Kooter, J. M., Matzke, M. A. & Meyer, P. Listening to the silent genes: transgene silencing, gene regulation and pathogen control. Trends Plant Sci. 4 , 340–347 (1999).
Venter, M. & Botha, F. C. in Plant Developmental Biology — Biotechnological Perspectives Vol. 2 (eds Pua, E. C. & Davey, M. R.) 393–408 (Springer, 2010).
Google Scholar
Liu, W., Mazarei, M., Rudis, M. R., Fethe, M. H. & Stewart, C. N. Jr. Rapid in vivo analysis of synthetic promoters for plant pathogen phytosensing. BMC Biotechnol. 11 , 108 (2011).
Liu, W. et al. Bacterial pathogen phytosensing in transgenic tobacco and Arabidopsis . Plant Biotechnol. J. 11 , 43–52 (2013). References 8 and 9 provide good examples of the use of synthetic promoters for the phytosensing of bacterial pathogen in plants.
Mehrotra, R. et al. Designer promoter: an artwork of cis engineering. Plant Mol. Biol. 75 , 527–536 (2011).
Hu, J., Li, B. & Kihara, D. Limitations and potentials of current motif discovery algorithms. Nucleic Acids Res. 33 , 4899–4913 (2005).
Tompa, M. et al. Assessing computational tools for the discovery of transcription factor binding sites. Nature Biotech. 23 , 137–144 (2005). This paper provides an excellent comparison of the effectiveness of 13 computational tools for de novo motif discovery.
CAS Google Scholar
Koschmann, J. et al. Intergration of bioinformatics and synthetic promoters leads to the discovery of novel elicitor-responsive cis -regulatory sequences in Arabidopsis . Plant Physiol. 160 , 178–191 (2012).
Mukherji, S. & van Oudenaarden, A. Synthetic biology: understanding biological design from synthetic circuits. Nature Rev. Genet. 10 , 859–871 (2009).
Sawant, S., Singh, P. K., Madanala, R. & Tuli, R. Designing of an artificial expression cassette for the high-level expression of transgenes in plants. Theor. Appl. Genet. 102 , 635–644 (2001).
Comai, L., Moran, P. & Maslyar, D. Novel and useful properties of a chimeric plant promoter combining CaMV 35S and MAS elements. Plant Mol. Biol. 15 , 373–381 (1990).
Petolino, J. F. & Davies, J. P. Designed transcriptional regulators for trait development. Plant Sci. 201–202 , 128–136 (2013).
Bogdanove, A. J. & Voytas, D. F. TAL effectors: customizable proteins for DNA targeting. Science 333 , 1843–1846 (2011).
Guan, X. et al. Heritable endogenous gene regulation in plants with designed polydactyl zinc finger transcription factors. Proc. Natl Acad. Sci. USA 99 , 13296–13301 (2010).
Sanchez, J.-P., Ullman, C., Moore, M., Choo, Y. & Chua, N.-H. Regulation of gene expression in Arabidopsis thaliana by artificial zinc finger chimeras. Plant Cell Physiol. 43 , 1465–1472 (2002).
Li, J. et al. Activation domains for controlling plant gene expression using designed transcription factors. Plant Biotechnol. J. 11 , 671–680 (2013).
Sanchez, J.-P., Ullman, C., Moore, M., Choo, Y. & Chua, N.-H. Regulation of Arabidopsis thaliana 4-coumarate: coenzyme-A ligase-1 expression by artificial zinc finger chimeras. Plant Biotechnol. J. 4 , 103–114 (2006).
Lindhout, B. I., Pinas, J. E., Hooykaas, P. J. J. & van der Zaal, B. J. Employing libraries of zinc finger artificial transcription factors to screen for homologous recombination mutants in Arabidopsis . Plant J. 48 , 475–483 (2006).
Holmes-Davis, R. et al. Gene regulation in planta by plant-derived engineered zinc finger protein transcription factors. Plant Mol. Biol. 57 , 411–423 (2005).
Van Eenennaam, A. L. et al. Elevation of seed α-tocopherol levels using plant-based transcription factors targeted to an endogenous locus. Metabol. Eng. 6 , 101–108 (2004).
Gupta, M. et al. Transcriptional activation of Brassica napus β-ketoacyl-ACP synthase II with an engineered zinc finger protein transcription factor. Plant Biotechnol. J. 10 , 783–791 (2012).
Ordiz, M. I., Magnenat, L., Barbas, C. F. 3rd. & Beachy, R. N. Negative regulation of the RTBV promoter by designed zinc finger proteins. Plant Mol. Biol. 72 , 621–630 (2010).
Moscou, M. J. & Bogdanove, A. J. A simple cipher governs DNA recognition by TAL effectors. Science 326 , 1501 (2009).
Boch, J. & Bonas, U. Xanthomonas AvrBs3 family-type III effectors: discovery and function. Annu. Rev. Phytopathol. 48 , 419–436 (2010).
Boch, J. et al. Breaking the code of DNA binding specificity of TAL-type III effectors. Science 326 , 1509–1512 (2009). References 28 and 30 are the two studies that first reported the molecular mechanisms of bacterial TALEs.
Morbitzer, R., Romer, P., Boch, J. & Lahaye, T. Regulation of selected genome loci using de novo -engineered transcription activator-like effector (TALE)-type transcription factors. Proc. Natl Acad. Sci. USA 107 , 21617–21622 (2010).
Mahfouz, M. M. et al. Targeted transcriptional repression using a chimeric TALE–SRDX repressor protein. Plant Mol. Biol. 78 , 311–321 (2012).
Knight, T. Idempotent vector design for standard assembly of Biobricks. DSpace@MIT [online] , (2003).
Anderson, J. C. et al. BglBricks: a flexible standard for biological part assembly. J. Biol. Eng. 4 , 1 (2010).
PubMed PubMed Central Google Scholar
Engler, C., Kandzia, R. & Marillonnet, S. A one pot, one step, precision cloning method with high throughput capability. PLoS ONE 3 , e3647 (2008).
Sasaki, Y. Evidence for high specificity and efficiency of multiple recombination signals in mixed DNA cloning by the MultiSite Gateway system. J. Biotechnol. 107 , 233 (2004).
Nour-Eldin, H. H., Geu-Flores, F. & Halkier, B. A. USER cloning and USER fusion: the ideal cloning techniques for small and big laboratories. Methods Mol. Biol. 643 , 185–200 (2010).
Sleight, S., Bartley, B., Lieviant, J. & Sauro, H. In-fusion BioBrick assembly and re-engineering. Nucleic Acids Res. 38 , 2624 (2010).
Li, M. Z. & Elledge, S. J. Harnessing homologous recombination in vitro to generate recombinant DNA via SLIC. Nature Methods 4 , 251–256 (2007).
Gibson, D. G. et al. Creation of a bacterial cell controlled by a chemically synthesized genome. Science 329 , 52–56 (2010). This study reports the largest de novo synthesized and assembled genome.
Hillson, N. J., Rosengarten, R. D. & Keasling, J. D. j5 DNA assembly design automation software. ACS Synth. Biol. 1 , 14–21 (2012).
Sarrion-Perdigones, A. et al. GoldenBraid: an iterative cloning system for standardized assembly of reusable genetic modules. PLoS ONE 6 , e21622 (2011).
Cardi, T., Lenzi, P. & Maliga, P. Chloroplasts as expression platforms for plant-produced vaccines. Expert Rev. Vaccines 9 , 893–911 (2010).
Scotti, N., Rigano, M. M. & Cardi, T. Production of foreign proteins using plastid transformation. Biotechnol. Adv. 30 , 387–397 (2012).
Maliga, P. & Bock, R. Plastid biotechnology: food, fuel, and medicine for the 21 st century. Plant Physiol. 155 , 1501–1510 (2011).
Clarke, J. L. & Daniell, H. Plastid biotechnology for crop production: present status and future perspectives. Plant Mol. Biol. 76 , 211–220 (2011).
Dufourmantel, N. et al. Generation and analysis of soybean plastid transformants expressing Bacillus thuringiensis Cry1 Abprotoxin. Plant Mol. Biol. 58 , 659–668 (2006).
Sidorov, V. A. et al. Stable chloroplast transformation in potato: use of green fluorescent protein as a plastid marker. Plant J. 19 , 209–216 (1999).
Apel, W. & Bock, R. Enhancement of carotenoid biosynthesis in transplastomic tomatoes by induced lycopene-to-provitamin A conversion. Plant Physiol. 151 , 59–66 (2009).
Lelivelt, C. L. C. et al. Stable plastid transformation in lettuce ( Lactuca sativa L.). Plant Mol. Biol. 58 , 763–774 (2005).
Kanamoto, H. et al. Efficient and stable transformation of Lactuca sativa L. cv. Cisco (lettuce) plastids. Transgen. Res. 15 , 205–217 (2006).
Marchis, F. D., Wang, Y., Stevanato, P., Arcioni, S. & Bellucci, M. Genetic transformation of the sugar beet plastome. Transgen. Res. 18 , 17–30 (2009).
Singh, A. K., Verma, S. S. & Bansal, K. C. Plastid transformation in eggplant ( Solanum melongena L.). Transgen. Res. 19 , 113–119 (2010).
Kumar, S., Dhingra, A. & Daniell, H. Plastid expressed betaine aldehyde dehydrogenase gene in carrot cultured cells, roots and leaves confers enhanced salt tolerance. Plant Physiol. 136 , 2843–2854 (2004).
Hou, B. K. et al. Chloroplast transformation in oilseed rape. Transgen. Res. 12 , 111–114 (2003).
Liu, C. W., Lin, C. C., Chen, J. J. & Tseng, M. J. Stable chloroplast transformation in cabbage ( Brassica oleracea L. var. Capitata L.) by particle bombardment. Plant Cell Rep. 26 , 1733–1744 (2007).
Werner, S., Breus, O., Symonenko, Y., Marillonnet, S. & Gleba, Y. High-level recombinant protein expression in transgenic plants by using a double-inducible viral vector. Proc. Natl Acad. Sci. USA 108 , 14061–14066 (2011).
Marton, I. et al. Nontransgenic genome modification in plant cells. Plant Physiol. 154 , 1079–1087 (2010).
Mozes-Koch, R. et al. Expression of an entire bacterial operon in plants. Plant Physiol. 158 , 1883–1892 (2012).
Liu, Y. G. et al. Complementation of plant mutants with large genomic DNA fragments by a transformation-competent artificial chromosome vector accelerates positional cloning. Proc. Natl Acad. Sci. USA 96 , 6535–6540 (1999).
Lin, L., Liu, Y.-G., Xu, X. & Li, B. Efficient linking and transfer of multiple genes by a multigene assembly and transformation vector system. Proc. Natl Acad. Sci. USA 100 , 5962–5967 (2003).
Hamilton, C. M., Frary, A., Lewis, C. & Tanksley, S. D. Stable transfer of intact high molecular weight DNA into plant chromosomes. Proc. Natl Acad. Sci. USA 93 , 9975–9979 (1996).
Chen, Q.-J. et al. MISSA is a highly efficient in vivo DNA assembly method for plant multiple-gene transformation. Plant Physiol. 153 , 41–51 (2010).
Ananiev, E. V. et al. Artificial chromosome formation in maize ( Zea mays L.). Chromosoma 118 , 157–177 (2009).
Carlson, S. R. et al. Meiotic transmission of an in vitro -assembled autonomous maize minichromosome. PLoS Genet. 3 , 1965–1974 (2007). This paper is the first report of the bottom-up approach for minichromosome generation in plants.
Kapusi, E. et al. Telomere-mediated truncation of barley chromosomes. Chromosoma 121 , 181–190 (2012).
Nelson, A. D., Lamb, J. C., Kobrossly, P. S. & Shippen, D. E. Parameters affecting telomere-mediated chromosomal truncation in Arabidopsis . Plant Cell 23 , 2263–2272 (2011).
Teo, C. H. et al. Induction of telomere-mediated chromosomal truncation and stability of truncated chromosomes in Arabidopsis thaliana . Plant J. 68 , 28–39 (2011).
Yu, W., Lamb, J. C., Han, F. & Birchler, J. A. Telomere-mediated chromosomal truncation in maize. Proc. Natl Acad. Sci. USA 103 , 17331–17336 (2006).
Yu, W., Han, F., Gao, Z., Vega, J. M. & Birchler, J. A. Construction and behavior of engineered minichromosomes in maize. Proc. Natl Acad. Sci. USA 104 , 8924–8929 (2007). References 69 and 70 are the first reports of the top-down approach for minichromosome generation in plants.
Frary, A. & Hamilton, C. M. Efficiency and stability of high molecular weight DNA transformation: an analysis in tomato. Transgen. Res. 10 , 121–132 (2001).
Houben, A., Dawe, R. K., Jiang, J. & Schubert, I. Engineered plant minichromosomes: a bottom-up success? Plant Cell 20 , 8–10 (2008).
Gaeta, R. T. & Krishnaswamy, L. in Plant Chromosome Engineering: Methods and Protocols, Methods in Molecular Biology Vol. 701 (ed. Birchler, J. A.) 131–146 (Springer, 2011).
Gaeta, R. T., Masonbrink, R. E., Krishnaswamy, L., Zhao, C. & Birchler, J. A. Synthetic chromosome platforms in plants. Annu. Rev. Plant Biol. 63 , 307–330 (2012).
Xu, C., Cheng, Z. & Yu, W. Construction of rice mini-chromosome by telomere-mediated chromosomal truncation. Plant J. 70 , 1070–1079 (2012).
Vega, J. M. et al. Agrobacterium -mediated transformation of maize ( Zea mays L.) with Cre –lox site specific recombination cassettes in BIBAC vectors. Plant Mol. Biol. 66 , 587–598 (2008).
Bibikova, M., Golic, M., Golic, K. G. & Carroll, D. Targeted chromosomal cleavage and mutagenesis in Drosophila using zinc-finger nucleases. Genetics 161 , 1169–1175 (2002). This is the first report of using ZFN for genome engineering by linking the cleavage domain of the Fok I restriction enzyme to designed ZFPs.
Porteus, M. H. & Baltimore, D. Chimeric nucleases stimulate gene targeting in human cells. Science 300 , 763 (2003).
Urnov, F. D. et al. Highly efficient endogenous human gene correction using designed zinc-finger nucleases. Nature 435 , 646–651 (2005).
Lloyd, A., Plaisier, C. L., Carroll, D. & Drews, G. N. Targeted mutagenesis using zinc-finger nucleases in Arabidopsis . Proc. Natl Acad. Sci. USA 102 , 2232–2237 (2005). This paper is the first report of the use of ZFNs in plants.
Christian, M. et al. Targeting DNA double-strand breaks with TAL effector nucleases. Genetics 186 , 757–761 (2010).
Li, T. et al. TAL nucleases (TALNs): hybrid proteins composed of TAL effectors and Fok I DNA-cleavage domain. Nucleic Acids Res. 39 , 359–372 (2010). References 81 and 82 are the first reports of the potential use of TALENs as site-specific endonucleases for selective genome cleavage.
Osakabe, K., Osakabe, Y. & Toki, S. Site-directed mutagenesis in Arabidopsis using custom-designed zinc finger nucleases. Proc. Natl Acad. Sci. USA 107 , 12034–12039 (2010).
Zhang, F. et al. High frequency targeted mutagenesis in Arabidopsis thaliana using zinc finger nucleases. Proc. Natl Acad. Sci. USA 107 , 12028–12033 (2010).
Townsend, J. A. et al. High-frequency modification of plant genes using engineered zinc-finger nucleases. Nature 459 , 442–445 (2009).
Shukla, V. K. et al. Precise genome modification in the crop species Zea mays using zinc-finger nucleases. Nature 459 , 437–443 (2009).
Curtin, S. J. et al. Targeted mutagenesis of duplicated genes in soybean with zinc-finger nucleases. Plant Physiol. 156 , 466–473 (2011).
Mahfouz, M. M. et al. De novo -engineered transcription activator-like effector (TALE) hybrid nuclease with novel DNA binding specificity creates double-strand breaks. Proc. Natl Acad. Sci. USA 108 , 2623–2628 (2011).
Zhang, Y. et al. Transcription activator-like effector nucleases enable efficient plant genome engineering. Plant Physiol. 161 , 20–27 (2013).
Cermak, T. et al. Efficient design and assembly of custom TALEN and other TAL effector-based constructs for DNA targeting. Nucleic Acids Res. 39 , e82 (2011).
Shan, Q. et al. Rapid and efficient gene modification in rice and Brachypodium using TALENs. Mol. Plant 6 , 1365–1368 (2013).
Li, T., Liu, B., Spalding, M. H., Weeks, D. P. & Yang, B. High-efficiency TALEN-based gene editing produces disease-resistant rice. Nature Biotech. 30 , 390–392 (2012).
De Pater, S., Neuteboom, L. W., Pinas, J. E., Hooykaas, P. J. J. & Van Der Zaal, B. J. ZFN-induced mutagenesis and gene-targeting in Arabidopsis through Agrobacterium -mediated floral dip transformation. Plant Biotechnol. J. 7 , 821–835 (2009).
Puchta, H., Dujon, B. & Hohn, B. Two different but related mechanisms are used in plants for the repair of genomic double-strand breaks by homologous recombination. Proc. Natl Acad. Sci. USA 93 , 5055–5060 (1996).
Gao, H. et al. Heritable targeted mutagenesis in maize using a designed endonuclease. Plant J. 61 , 176–187 (2010).
D'Halluin, K., Vanderstraeten, C., Stals, E., Cornelissen, M. & Ruiter, R. Homologous recombination: a basis for targeted genome optimization in crop species such as maize. Plant Biotechnol. J. 6 , 93–102 (2008).
Yang, M. et al. Targeted mutagenesis in the progeny of maize transgenic plants. Plant Mol. Biol. 70 , 669–679 (2009).
Prieto, J. et al. The C terminal loop of the homing endonuclease I Cre I is essential for site recognition, DNA binding and cleavage. Nucleic Acids Res. 35 , 3262–3271 (2007).
Haurwitz, R. E., Jinek, M., Wiedenheft, B., Zhou, K. & Doudna, J. A. Sequence- and structure-specific RNA processing by a CRISPR endonuclease. Science 329 , 1355–1358 (2010).
Deltcheva, E. et al. CRISPR RNA maturation by trans -encoded small RNA and host factor RNase III. Nature 471 , 602–607 (2011).
Jinek, M. et al. A programmable dual-RNA-Guided DNA endonuclease in adaptive bacterial immunity. Science 337 , 816–821 (2012). The first report that the engineered fusion of mature crRNAs to tracrRNA forms a two-RNA structure which directs dual RNA-guided genome editing.
Li, J.-F. et al. Multiplex and homologous recombination-mediated genome editing in Arabidopsis and Nicotiana benthamiana using guide RNA and Cas9. Nature Biotech. 31 , 688–691 (2013).
Nekrasov, V., Staskawicz, B., Weigel, D., Jones, J. D. G. & Kamoun, S. Targeted mutagenesis in the model plant Nicotiana benthamiana using Cas9 RNA-guided endonuclease. Nature Biotech. 31 , 691–693 (2013).
Shan, Q. et al. Targeted genome modification of crop plants using a CRISPR–Cas system. Nature Biotech. 31 , 686–688 (2013). References 102–104 are the first reports of the application of the CRISPR–Cas system for targeted genome modification in plants.
Daniell, H. Molecular strategies for gene containment in transgenic crops. Nature Biotech. 20 , 581–586 (2002).
Yau, Y. Y. & Stewart, C. N. Jr. Less is more: strategies to remove marker genes from transgenic plants. BMC Biotechnol. 13 , 36 (2013).
Akbudak, M. A. & Srivastava, V. Improved FLP recombinase, FLPe, efficiently removes marker gene from transgene locus developed by Cre– lox mediated site-specific gene integration in rice. Mol. Biotechnol. 49 , 82–89 (2011).
Khan, R. S., Nakamura, I. & Mii, M. Development of disease-resistant marker-free tomato by R/ RS site-specific recombination. Plant Cell Rep. 30 , 1041–1053 (2011).
Lloyd, A. M. & Davis, R. W. Functional expression of the yeast FLP/ FRT site-specific recombination system in Nicotiana tabacum . Mol. Gen. Genet. 242 , 653–657 (1994).
Luo, K. et al. 'GM-gene-deletor': fused loxP – FRT recognition sequences dramatically improve the efficiency of FLP or CRE recombinase on transgene excision from pollen and seed of tobacco plants. Plant Biotechnol. J. 5 , 263–274 (2007).
Nandy, S. & Srivastava, V. Site-specific gene integration in rice genome mediated by the FLP– FRT recombination system. Plant Biotechnol. J. 9 , 713–721 (2011).
Kolodii, G. The shuffling function of resolvases. Gene 269 , 121–130 (2001).
Rao, M. R. et al. FLP/ FRT recombination from yeast: application of a two gene cassette scheme as an inducible system in plants. Sensors 10 , 8526–8535 (2010).
Zuo, J., Niu, Q. W., Moller, S. G. & Chua, N.-H. Chemical-regulated, site-specific DNA excision in transgenic plants. Nature Biotech. 19 , 157–161 (2001).
Thomson, J. G. & Ow, D. W. Site-specific recombination systems for the genetic manipulation of eukaryotic genomes. Genesis 44 , 465–476 (2006).
Wang, Y., Yau, Y. Y., Perkins-Balding, D. & Thomson, J. G. Recombinase technology: applications and possibilities. Plant Cell Rep. 30 , 267–285 (2011).
Thomason, L. C., Calendar, R. & Ow, D. W. Gene insertion and replacement in Schizosaccharomyces pombe mediated by the Streptomyces bacteriophage phiC31 site-specific recombination system. Mol. Genet. Genomics 265 , 1031–1038 (2001).
Moon, H. S. et al. Transgene excision in pollen using a codon optimized serine resolvase CinH– RS2 site-specific recombination system. Plant Mol. Biol. 75 , 621–631 (2011).
Petolino, J. F. et al. Zinc finger nuclease-mediated transgene deletion. Plant Mol. Biol. 73 , 617–628 (2010).
Zhou, Y., Yau, Y.-Y., Ow, D. W. & Wang, Y. Site-specific deletions in the tomato genome by the CinH– RS2 and ParA– MRS recombination systems. Plant Biotechnol. Rep. 6 , 225–232 (2012).
Thomson, J. G. et al. ParA resolvase catalyzes site-specific excision of DNA from the Arabidopsis genome. Transgen. Res. 18 , 237–248 (2009).
Thomson, J. G. et al. The Bxb1 recombination system demonstrates heritable transmission of site-specific excision in Arabidopsis . BMC Biotechnol. 12 , 9 (2012).
Thomson, J. G., Chan, R., Thilmony, R., Yau, Y.-Y. & Ow, D. W. PhiC31 recombination system demonstrates heritable germinal transmission of site-specific excision from the Arabidopsis genome. BMC Biotechnol. 10 , 17 (2010).
Antunes, M. S., Smith, J. J., Jantz, D. & Medford, J. I. Targeted DNA excision in Arabidopsis by a re-engineered homing endonuclease. BMC Biotechnol. 12 , 86 (2012).
Blechl, A., Lin, J., Shao, M., Thilmony, R. & Thomson, J. The Bxb1 recombinase mediates site-specific deletion in transgenic wheat. Plant Mol. Biol. Rep. 30 , 1357–1366 (2012).
Kempe, K. et al. Transgene excision from wheat chromosome by phage phiC31 integrase. Plant Mol. Biol. 72 , 673–387 (2010).
Sang, Y., Millwood, R. J. & Stewart, C. N. Jr. Gene use restriction technologies for transgenic plant bioconfinement. Plant Biotechnol. J. 11 , 649–658 (2013).
Schwille, P. Bottom-up synthetic biology: engineering in a tinkerer's world. Science 333 , 1252–1254 (2011).
Antunes, M. S. et al. Programmable ligand detection system in plants through a synthetic signal transduction pathway. PLoS ONE 6 , e16292 (2011).
Borak, B., Ort, D. R. & Burbaum, J. J. Energy and carbon accounting to compare bioenergy crops. Curr. Opin. Biotechnol. 24 , 369–375 (2013).
Bradford, K. J. et al. Regulating transgenic crops sensibly: lessons from plant breeding, biotechnology and genomics. Nature Biotech. 23 , 439–444 (2005).
Dhar, M. K., Kaul, S. & Kour, J. Towards the development of better crops by genetic transformation using engineered plant chromosomes. Plant Cell Rep. 30 , 799–806 (2011).
Hirano, N. et al. Site-specific recombination system based on actinophage TG1 integrase for gene integration into bacterial genomes. Appl. Microbiol. Biotechnol. 89 , 1877–1884 (2011).
Ghosh, P., Pannunzio, N. R. & Hatfull, G. F. Synapsis in phage Bxb1 integration: selection mechanism for the correct pair of recombination sites. J. Mol. Biol. 349 , 331–348 (2005).
Blake, W. et al. Pairwise selection assembly for sequence-independent construction of long-length DNA. Nucleic Acids Res. 38 , 2594 (2010).
Cha-aim, K., Fukunaga, T., Hoshida, H. & Akada, R. Reliable fusion PCR mediated by GC-rich overlap sequences. Gene 434 , 43–49 (2009).
Quan, J. & Tian, J. Circular polymerase extension cloning of complex gene libraries and pathways. PLoS ONE 4 , e6441 (2009).
Download references
Acknowledgements
The authors thank J. Haseloff, who provided much feedback on earlier drafts. They appreciate their respective universities for the freedom and resources to undertake this Review, including funding by Advanced Research Projects Agency-Energy to J.S.Y. and by the BioEnergy Science Center to C.N.S. The BioEnergy Science Center is a US Department of Energy (DOE) Bioenergy Research Center supported by the Office of Biological and Environmental Research in the DOE Office of Science. The authors appreciate the work of multiple anonymous reviewers. They thank J. Hinds and M. Rudis for their assistance on various drafts of the paper.
Author information
Authors and affiliations.
Department of Plant Sciences, University of Tennessee, Knoxville, 37996, Tennessee, USA
Wusheng Liu & C. Neal Stewart Jr
Department of Plant Pathology and Microbiology, Texas A&M AgriLife Synthetic and Systems Biology Innovation Hub, Institute of Plant Genomics and Microbiology, Texas A&M University, College Station, 77843, Texas, USA
Joshua S. Yuan
BioEnergy Science Center, Oak Ridge National Laboratory, Oak Ridge, 37831, Tennessee, USA
C. Neal Stewart Jr
You can also search for this author in PubMed Google Scholar
Corresponding author
Correspondence to C. Neal Stewart Jr .
Ethics declarations
Competing interests.
J.S.Y. is a principal in a start-up company. C.N.S.Jr and J.S.Y. have received grants and contracts from the private sector, which has an interest in plant biotechnology.
Related links
Further information.
Plant cis -acting regulatory DNA elements database
Synbio.org — plants
Synthetic biology
Systems-Biology
PowerPoint slides
Powerpoint slide for fig. 1, powerpoint slide for fig. 2, powerpoint slide for fig. 3, powerpoint slide for fig. 4, powerpoint slide for fig. 5, powerpoint slide for table 1, supplementary information, supplementary information s1 (table).
Synthetic promoters that have been designed for the regulation of transgene expression in plants (PDF 227 kb)
The most common plant transformation method. It involves the transfer of genes of interest from A. tumefaciens vectors and the subsequent integration of these genes into plant nuclear genomes.
A commonly used transformation method in which high velocity microprojectiles coated with gene constructs are used to deliver genes into cells and tissues.
The fusion of engineered DNA-binding proteins or domains with sequence specificities to effector domains that modify genetic sequences and/or gene expression.
Genome modification achieved by the induction of a double-strand break in a specific genome site, followed by DNA-break repair and the generation of desired modifications (gene disruption, addition or correction).
A gene silencing phenomenon induced by homologous sequences at the transcriptional or post-transcriptional levels.
(ZFPs). DNA-binding protein domains that consist of a tandem array of 2–9 zinc-fingers, each of which recognizes approximately three bases of DNA sequence.
(TALEs). Major virulence factors (containing an amino terminus, a unique type of central DNA-binding domain and a carboxyl terminus with the activation domain) that are secreted by the pathogenic Xanthomonas spp. bacterium when it infects plants. Their DNA-binding domains can be custom-designed to specifically bind to any DNA sequences.
The precise joining of DNA fragments without the addition of intervening or unwanted nucleotides at the junctions.
The synthesis of continuous strands of DNA molecules using a laboratory instrument without the presence of pre-existing templates.
An engineered non-integrating vector that harbours large amounts of DNA (including telomeres, origins of replication, a centromere and genes of interest) and is transmissible in cell division after transformation into plant cells.
A site-specific recombination system mediated by the Cre recombinase in a genome that contains pre-existing or pre-engineered loxP sites which are recognized by the Cre recombinase.
The accumulation of multiple trangenes of interest into the same plant genome for stacked traits.
A recombination system in which the FLP recombinase specifically recognizes the FRT site and mediates excision of any sequence that is flanked by the FRT sites.
Supernumerary or accessory chromosomes that are heterochromatic. They do not contain functional genes and do not to pair with A chromosomes at meiosis.
(ZFNs). Fusions of engineered zinc-finger arrays (that consist of 3–6 C2H2 fingers) to a non-specific DNA-cleavage domain of the Fok I endonuclease.
(TALENs). Fusions of truncated TALEs (containing an amino terminus, a custom-designed DNA-binding domain and a carboxyl terminus with the activation domain being removed) to a non-specific DNA-cleavage domain of the Fok I endonuclease.
The incorporation of a transgene (or transgenes) of interest into one or more desired specific genomic loci for the permanent modification of plant genomes using homologous recombination (or another method) followed by the selection for a rare recombination event.
The most common transient transformation method in plants which uses injection or vacuum infiltration to transform genes into cells and tissues using Agrobacterium tumefaciens .
The replacement of normal sexual reproduction by asexual reproduction without fertilization.
A phenomenon in which certain plants propagate using non-opening, self-pollinating flowers.
Rights and permissions
Reprints and permissions
About this article
Cite this article.
Liu, W., Yuan, J. & Stewart Jr, C. Advanced genetic tools for plant biotechnology. Nat Rev Genet 14 , 781–793 (2013). https://doi.org/10.1038/nrg3583
Download citation
Published : 09 October 2013
Issue Date : November 2013
DOI : https://doi.org/10.1038/nrg3583
Share this article
Anyone you share the following link with will be able to read this content:
Sorry, a shareable link is not currently available for this article.
Provided by the Springer Nature SharedIt content-sharing initiative
This article is cited by
An orthogonalized pyr1-based cid module with reprogrammable ligand-binding specificity.
- Sang-Youl Park
- Sean R. Cutler
Nature Chemical Biology (2024)
Heterologous mogrosides biosynthesis in cucumber and tomato by genetic manipulation
- Jingjing Liao
- Tingyao Liu
Communications Biology (2023)
Genetic engineering of plants for phytoremediation: advances and challenges
- Kundan Kumar
- Akshay Shinde
- Naseera Sayed Arif
Journal of Plant Biochemistry and Biotechnology (2023)
Quick links
- Explore articles by subject
- Guide to authors
- Editorial policies
Sign up for the Nature Briefing: Translational Research newsletter — top stories in biotechnology, drug discovery and pharma.


IMAGES
COMMENTS
Discover the latest research and news on plant biotechnology from Nature Portfolio, covering topics such as genetic engineering, tissue culture and biofuels.
Plant biotechnology is a mature technology. Modern biotechnology was a scientifically obvious outcome of the striking advances in molecular biology that followed the discovery of the bacterial DNA restriction-modification system (Luria and Human, 1952; Luria, 1953; Dussoix and Arber, 1962; Nathans and Smith, 1975).Microorganisms and plants were the first organisms to be manipulated to serve ...
Plant biotechnology articles within Nature Biotechnology. Featured. Research Briefing | 20 May 2024. Advancing programmable gene expression in plants using CRISPRi-based Boolean gates.
Recent advances in crop transformation technologies. Zongliang Chen, Juan M. Debernardi, Jorge Dubcovsky &. Andrea Gallavotti. Nature Plants 8 , 1343-1351 ( 2022) Cite this article. 8152 ...
Plant biotechnology has gained importance in the recent past for augmenting the quality and quantity of agricultural, horticultural, ornamental plants, and in manipulating the plants for improved agronomic performance. Plant tissue culture is the most popular technique of plant biotechnology, which has diverse applications in the various fields.
Plant Biotechnology Journal (PBJ) is an open access journal publishing high-impact original research and incisive reviews with an emphasis on molecular plant sciences and their applications through plant biotechnology.It is published by Wiley in collaboration with the Society for Experimental Biology (SEB) and the Association of Applied Biologists (AAB).
Plant Biotechnology Journal is published by Wiley-Blackwell in association with the Society for Experimental Biology (SEB) and the Association of Applied Biology (AAB). Plant Biotechnology Journal aims to publish high-impact original research and incisive reviews by leading researchers in applied plant science, with an emphasis on molecular plant sciences and their applications through plant ...
Applications of Fast Breeding Technologies in Crop Improvement and Functional Genomics Study. Xingguo Ye. James A. Birchler. Fangpu Han. 24,623 views. 16 articles. This section explores all branches of plant biotechnology, addressing the attempts of modern technologies to satisfy increasing demands for crop production.
Plant Biotechnology Reports is a peer-reviewed journal emphasizing fundamental and applied research in plant biotechnology. Offers comprehensive coverage extending to molecular biology, genetics, biochemistry, and more. Prioritizes studies on plants indigenous to the Asia-Pacific region. Encourages studies related to commercialization of plant ...
The Plant Biotechnology section at Frontiers in Plant Science mainly publishes applied studies examining how plants can be improved using modern genetic techniques (Lloyd and Kossmann, 2021). This Research Topic was designed to allow editors from the section to highlight some of their own plant biotechnological work.
Scope. Plant Biotechnology publishes significant findings and major advances in all branches of plant biotechnology, providing a single platform for articles that address the attempts of modern technologies to satisfy increasing demands for crop production and to extend the exploitability of plants to include other sustainable uses.
Plant biotechnology is a set of techniques used to adapt plants for specific needs or opportunities. Situations that combine multiple needs and opportunities are common. For example, a single crop may be required to provide sustainable food and healthful nutrition, protection of the environment, and opportunities for jobs and income. Finding or developing suitable plants is typically a highly ...
Research fields, including biotechnology, functional genomics, precision plant breeding, and disease diagnostics benefited from the applications of metabolomics and also the move forward toward translational metabolomics . Recent advancements have sped the screening process, and the incorporation of metabolomic technologies will shorten the ...
Here, we reviewed challenges in plant bioengineering and discussed opportunities in the emerging field of nano-based plant biotechnology to advance plant genetic engineering, as well as post ...
Main body. Particularly in plant biotechnology, the amount of information has multiplied exponentially with a large number of databases available from many individual plant species. Efficient bioinformatics tools and methodologies are also developed to allow rapid genome sequence and the study of plant genome in the 'omics' approach.
Plant biotechnology applies to three major areas of plants and their uses: (1) control of plant growth and development; (2) protection of plants against biotic and abiotic stresses; and (3) expansion of ways by which specialty foods, biochemicals, and pharmaceuticals are produced. ... Plant Protection Research Unit, U.S. Plant, Soil, and ...
Plant Tissue Culture-Based Industries. Saurabh Bhatia, Kiran Sharma, in Modern Applications of Plant Biotechnology in Pharmaceutical Sciences, 2015. 14.1 Introduction. Plant biotechnology is a powerful tool for the development of new plant traits and varieties. Such new varieties must be produced on a large scale to achieve commercial success and to satisfy the demand from growers.
Plant Biotechnology Journal (PBJ) is an open access journal publishing high-impact original research and incisive reviews with an emphasis on molecular plant sciences and their applications through plant biotechnology.It is published by Wiley in collaboration with the Society for Experimental Biology (SEB) and the Association of Applied Biologists (AAB).
Plant Biotechnology. Plant Biotechnology. Published by Japanese Society for Plant Biotechnology. 2,223 registered articles (updated on April 17, 2022) Online ISSN : 1347-6114 Print ISSN : 1342-4580 ISSN-L : 1342-4580. Journal home.
She investigates their role in gene expression regulation and plant development, with implications for biotechnology in hybrid plant improvement. At the core of Patricia's research is the quest to unravel the mechanisms governing gene expression regulation and plant development through the lens of small RNAs.
Plant biology is a key area of science that bears major weight in the mankind's ongoing and future efforts to combat the consequences of global warming, climate change, pollution, and population growth. An in-depth understanding of plant physiology is paramount to our ability to optimize current agricultural practices, to develop new crop ...
Research Briefing | 20 May 2024. Advancing programmable gene expression in plants using CRISPRi-based Boolean gates
Oil palm is a versatile oil crop with numerous applications. Significant progress has been made in applying histological techniques in oil palm research in recent years. Whole genome sequencing of oil palm has been carried out to explain the function and structure of the order genome, facilitating the development of molecular markers and the construction of genetic maps, which are crucial for ...
Protein making and protein folding capacity must be in balance, like a sous chef and a chef, working in tandem. If the sous chef is providing the chef with too little or too many ingredients, it ...
The Business Research Company's Plant Genomics Global Market Report 2024 - Market Size, Trends, And Global Forecast 2024-2033 ... Biotechnology News Today by EIN Newsdesk & EIN Presswire (a press release distribution service) Follow us on Facebook & Twitter and connect with us on LinkedIn. Newsmatics Inc., 1025 ...
Plant sciences articles from across Nature Portfolio. ... Plant biotechnology; Plant breeding; Plant cell biology; ... Research Open Access 08 Aug 2024 Nature Ecology & Evolution. P: 1-13 ...
In addition to the gift from Albaum and Cerino, the Morris is expanding its focus on native plants in other ways. They are set to break ground on a new plant science lab—a fundraising priority—in the fall of 2024, which will focus on regionally rare plant species and forest health. "The Morris has long been a leader in botanical science ...
1 Key Laboratory of Engineering Biology for Low-carbon Manufacturing, Tianjin Institute of Industrial Biotechnology, Chinese Academy of Sciences, Tianjin, China; 2 University of Chinese Academy of Sciences, Beijing, China; 3 Tobacco Research Institute, Chinese Academy of Agricultural Sciences, Qingdao, China; 4 Govind Ballabh Pant National Institute of Himalayan Environment, Almora, India
New tools for plant biotechnology are emerging, including synthetic promoters, 'tunable' transcription factors, genome-editing tools and site-specific recombinases. These tools promise to expand ...
Research Research ... Specific areas of interest include health and human rights, biotechnology, reproduction, gender and health, mental health, drug and alcohol law and policy, medical negligence, doctor-patient relationships, occupational health and safety, healthcare and the criminal law, injury and disability law and compensation systems. ...